
Search by keywords or author
- Nano-Micro Letters
- Vol. 16, Issue 1, 143 (2024)
References
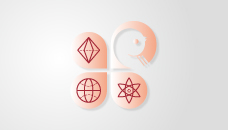
Jie Ma, Siyang Xing, Yabo Wang, Jinhu Yang, Fei Yu. Kinetic-Thermodynamic Promotion Engineering toward High-Density Hierarchical and Zn-Doping Activity-Enhancing ZnNiO@CF for High-Capacity Desalination[J]. Nano-Micro Letters, 2024, 16(1): 143
Download Citation
Set citation alerts for the article
Please enter your email address