
Search by keywords or author
- Nano-Micro Letters
- Vol. 15, Issue 1, 192 (2023)
References
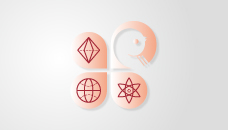
Changsheng Ding, Zhang Chen, Chuanxiang Cao, Yu Liu, Yanfeng Gao. Advances in Mn-Based Electrode Materials for Aqueous Sodium-Ion Batteries[J]. Nano-Micro Letters, 2023, 15(1): 192
Download Citation
Set citation alerts for the article
Please enter your email address