Abstract
We experimentally and theoretically demonstrate that the property (odd or even) of generated harmonics can be selected by manipulating the macroscopic phase-matching conditions based on a three-color laser field. Only odd or even harmonics can be made dominant by changing the focal position and adjusting the gas pressure. These results indicate that the odd-even property of the generated harmonics can be controlled by using the multi-color laser field with macroscopic phase-matching.High harmonic generation (HHG) in rare gases has been intensively studied over the past decades[1–6], and the process of HHG in the gas medium has been well understood and well described by a semi-classical three-step model[7,8]. Since the first experimental demonstration of harmonic generation[9], the conversion efficiency has been considerably enhanced[10–13]. This progress is due to a better understanding of both the microcosmic and macroscopic aspects, i.e. the atomic response[14] and the macroscopic control of phase-matching conditions[15]. As an effective scheme for enhancing the yield of a harmonic generation, the application of a multi-color laser field for HHG has been an interesting topic. As we all know, the harmonic spectrum only consists of the odd harmonics, due to the inversion symmetry in the fundamental laser field. However, research on harmonic generation in a two-color laser field presented not only a significant enhancement of the harmonic signal, but also the generation of the even harmonics, due to the symmetry breaking of the Hamiltonian by the presence of the additional weak field[16–18]. Harmonics are usually emitted in the form of an extreme ultraviolet (XUV) frequency comb with similar intensities in the plateau region due to their non-perturbative nature. The selective generation of a single harmonic emission from the comb can be implemented based on the phase-matching effect[19–23]. This is very important for some applications, such as the seeding of an XUV free-electron laser for obtaining a fully coherent output[24]. Our previous works[21–23] were mainly aimed at increasing the peak intensity and the contrast ratio (i.e. the spectral purity) as much as possible. Beyond that, the rules and selections of the harmonic property (odd or even) are also very important for better understanding and controlling the single harmonic emission.
In this Letter, we theoretically and experimentally investigate the rules and selections of the odd-even property for a single harmonic in argon based on a three-color laser field. The three-color laser field is synthesized using the fundamental, second, and third harmonic of an 800 nm multi-cycle Ti:sapphire laser pulse. Our demonstration indicates that the property (odd or even) of the harmonic emission can be made dominant by changing the focal position, and that the number of harmonic orders can be selected by adjusting the gas pressure.
The schematic of the experimental setup is shown in Fig. 1. A commercial Ti:sapphire femtosecond laser (Coherent, Inc.) is used to produce 2 mJ laser pulses at an 800 nm center wavelength with a 45 fs (full width at half maximum, FWHM) pulse duration at a repetition rate of 1 kHz. The pulses are directed into the vacuum chamber for harmonic generation. In the vacuum chamber, the laser beam is collimated and sequentially passes through a lens (with a focal length of 1100 mm), a SH-BBO crystal (-barium borate crystal, , , 0.3 mm thickness, type I phase-matching), a crystal (, , 0.4 mm thickness), and a TH-BBO crystal (-barium borate crystal, , , 0.1 mm thickness, type II phase-matching). It is then focused into a long argon gas cell with a length of 20 mm. The SH-BBO crystal is used for the second harmonic generation, and the polarization of the generated 400 nm pulse is perpendicular to that of the fundamental 800 nm pulse. The crystal is used for controlling the time delay between the 400 and 800 nm pulses. The TH-BBO is used for the third harmonic generation, and the polarization of the generated 267 nm pulse is parallel to that of the fundamental 800 nm pulse. In addition, a diagram with a diameter of approximately 10 mm is used in front of the lens (outside of the vacuum chamber) for modifying the laser beam profile. The parameters of the three-color laser field are the same as the ones reported in our previous work[22]. Finally, the generated harmonics are detected by a homemade flat-field grating spectrometer with a soft X-ray CCD (Princeton Instruments, SX 400). A 500 nm thick aluminum foil is used to block the driving laser in the front of the spectrometer.
Sign up for Chinese Optics Letters TOC. Get the latest issue of Chinese Optics Letters delivered right to you!Sign up now
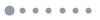
Figure 1.Schematic of the experimental setup.
Using the scheme mentioned above, we can optimize the harmonic signal by modifying the laser beam profile, changing the focal position, adjusting the gas pressure, and controlling the time delays among the different colors. The symmetry breaking that precisely originates from the superposition of the multi-color laser field can give rise to the generation of even harmonics. Through testing, we found that the property (odd or even) of the harmonic emission is mainly affected by changing the focal position and adjusting the gas pressure. The experimentally measured harmonic spectra with different focal positions are shown in Fig. 2, where the gas pressure is fixed to 10 torr for obtaining the optimum harmonic intensity. One can find that the intensity of the even harmonic (14th) has dramatically decreased (while the intensity of the odd harmonics has slowly decreased) when the focal position changes from 4 mm (the laser beam is focused before the center of the gas cell) to (the laser beam is focused after the center of the gas cell). In other words, the even harmonics are dominant in the harmonic spectrum at the focal position of 4 mm, as shown in Fig. 2(a), while the odd harmonics gradually become dominant in the harmonic spectrum at the focal position of , as shown in Fig. 2(c). This result indicates that the property (odd or even) of the harmonic emission can be affected by changing the focal position.
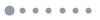
Figure 2.Experimentally measured harmonic spectra with different focal positions. The argon gas pressure is fixed at 10 torr.
We also observe the variation of the harmonic spectrum with different gas pressures at two particular focal positions. The experimentally measured harmonic spectra with different gas pressures are shown in Fig. 3, where the focal position is fixed to 4 mm to obtain the dominant even harmonics shown in Figs. 3(a)–3(c), and fixed to for obtaining the dominant odd harmonics shown in Figs. 3(d)–3(f). One can find that the purity of the even harmonics shown in Figs. 3(a)–3(c) first decreases and then increases (while the purity of the odd harmonics shown in Figs. 3(d)–3(f) first increases and then decreases) when the gas pressure changes from 2.5 to 80 torr. This result indicates that the change rule of the odd-even harmonics is very different, and that the number of harmonic orders is mainly affected by the variation of the gas pressure.
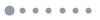
Figure 3.Experimentally measured harmonic spectra with different gas pressures at the two focal positions of 4 mm (the top row) and (the bottom row).
Finally, we experimentally optimize the laser beam profile, the focal position, the gas pressure, and the time delays for the selective generation of only odd or even harmonics. The optimum experimental results are shown in Figs. 4(a) and 4(b). In Fig. 4(a), one can see that only one even harmonic (14th) is selectively generated, while the other harmonics are dramatically suppressed when the gas pressure is adjusted to 80 torr at the focal position of 16 mm. Similarly, as shown in Fig. 4(b), only one or two odd harmonics (17th and 15th) are selectively generated, while the other harmonics are almost completely suppressed when the gas pressure is adjusted to 10 torr at the focal position of .
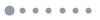
Figure 4.Top: Experimentally optimized results for (a) only even harmonics and (b) only odd harmonics. Bottom: Theoretically optimized results for (c) only even harmonics and (d) only odd harmonics.
We also theoretically generated only odd or even harmonics based on the Strong Field Approximation method[25,26], including the macroscopic propagation effect[27]. We used an iterative algorithm to search for the optimum results, because a large number of parameters must be considered in numerical calculations based on the three-color scheme. Finally, a set of appropriate parameters is obtained. The laser intensity ratios among the three colors are considered and are consistent with the experimental conditions. In the simulation, all three laser pulses are assumed to be Gaussian both in the temporal and spatial domains, with a pulse duration of 45 fs (FWHM), and a peak intensity of for 800 nm, for 400 nm, and for 267 nm. The length of the interaction gas medium is 20 mm, which is consistent with that of the gas cell in the experiment. The focal position is changed from to 20 mm, and the gas pressure is adjusted from 2.5 to 120 torr. The approximate results are obtained, and are shown in Figs. 4(c) and 4(d). In Fig. 4(c), when the focal position is changed to and the gas pressure is adjusted to 10 torr, only one even harmonic (12th) is selectively generated, while the other harmonics are dramatically suppressed. Similarly, as shown in Fig. 4(d), when the focal position is changed to and the gas pressure is adjusted to 20 torr, only one odd harmonic (13th) is obviously selectively generated, while all of the other harmonics are suppressed.
In our multi-color laser field, the phase matching of the harmonic emission can be affected by many parameters, such as the gas pressure, the interaction geometry, the spatial mode, the laser intensity, the intensity ratios among the different colors, the relative delays and the linear chirps of the multi-color laser field, and so on. The proper selection of these parameters will enable the harmonic emission to be phase-matched and confined to some particular orders. In our experiment, we think the main contributions of the phase matching are the neutral dispersion and the focusing geometry, which can be controlled by adjusting the gas pressure and changing the focal position. The focusing geometry can dominate the odd or even property, while the neutral dispersion can select the single harmonic.
For a gaseous medium[15], the phase mismatch resulting from the neutral and plasma can be compensated partly by varying the gas pressure. Moreover, the focusing geometry can induce the axial variation of the laser intensity and the variation of the Gouy phase. The dipole phase, which is dependent on the laser field intensity, has important consequences for phase matching[28]. The intensity dependence of the dipole phase can be understood from the semi-classical model[25]. The contribution from the Gouy phase can be controlled by changing the focal position with respect to the center of the gas cell. This change in the phase-matching conditions influences the spectral features of the harmonic emission[29]. In our experiment, changing the focal position would influence the dipole phase and the Gouy phase. When the appropriate focusing geometry (i.e. the appropriate focal position) is achieved for different harmonic orders, the odd or even harmonics can be selectively generated.
Therefore, the particular phase-matching conditions for only even or odd harmonics can be obtained by changing the focal position and adjusting the gas pressure. Although the simulated results have not well reproduced the experimental results, the numerical simulation does show that only even or odd harmonics can be selectively generated by using the multi-color laser field with the optimum macroscopic phase-matching conditions. Here, we only give some qualitative interpretations. The precise quantitative explanation for phase matching needs a more complex numerical simulation.
In conclusion, we experimentally and theoretically investigate the odd-even property of the generated harmonics based on a three-color laser field with macroscopic phase-matching conditions, and find that the odd-even property can be made dominant by changing the focusing geometry, while the number of harmonic orders can be selected by adjusting the gas pressure. These investigations are very important for better understanding and controlling the odd-even property of harmonic emission.
References
[1] M. Hentschel, R. Kienberger, Ch. Spielmann, G. A. Reider, N. Milosevic, T. Brabec, P. Corkum, U. Heinzmann, M. Drescher, F. Krausz. Nature (London), 414, 509(2001).
[2] P. Zhang, Y. Song, Z. Zhang. Phys. Rev. A, 78, 013811(2008).
[3] S. Tang, X. Chen. Phys. Rev. A, 82, 013827(2010).
[4] L. Shi, W. Li, H. Zhou, D. Wang, L. Ding, H. Zeng. Phys. Rev. A, 88, 053825(2013).
[5] J. Miao, P. Wei, Z. Zeng, C. Li, X. Ge, R. Li, Z. Xu. Chin. Opt. Lett., 12, 040201(2014).
[6] C. Liu, P. Wei, J. Miao, C. Zhang, Y. Huang, Y. Zheng, Y. Leng, Z. Zeng. Chin. Opt. Lett., 12, 030201(2014).
[7] P. B. Corkum. Phys. Rev. Lett., 71, 1994(1993).
[8] K. J. Schafer, B. Yang, L. F. DiMauro, K. C. Kulander. Phys. Rev. Lett., 70, 1599(1993).
[9] L. A. Lompré, A. L’Huillier, M. Ferray, P. Monot, G. Mainfray, C. Manus. J. Opt. Soc. Am. B, 7, 754(1990).
[10] A. L’Huillier, Ph. Balcou. Phys. Rev. Lett., 70, 774(1993).
[11] S. Kazamias, D. Douillet, F. Weihe, C. Valentin, A. Rousse, S. Sebban, G. Grillon, F. Augé, D. Hulin, Ph. Balcou. Phys. Rev. Lett., 90, 193901(2003).
[12] I. Kim, C. Kim, H. Kim, G. Lee, Y. Lee, J. Park, D. Cho, C. Nam. Phys. Rev. Lett., 94, 243901(2005).
[13] L. Brugnera, F. Frank, D. J. Hoffmann, R. Torres, T. Siegel, J. G. Underwood, E. Springate, C. Froud, E. I. C. Turcu, J. W. G. Tisch, J. P. Marangos. Opt. Lett., 35, 3994(2010).
[14] M. Lewenstein, Ph. Balcou, M. Yu. Ivanov, A. L’Huillier, P. B. Corkum. Phys. Rev. A, 49, 2117(1994).
[15] A. Rundquist, C. G. Durfee, Z. Chang, C. Herne, S. Backus, M. M. Murnane, H. C. Kapteyn. Science, 280, 1412(1998).
[16] E. Cormier, M. Lewenstein. Eur. Phys. J. D, 12, 227(2000).
[17] M. D. Perry, J. K. Crane. Phys. Rev. A, 48, R4051(1993).
[18] H. Eichmann, A. Egbert, S. Nolte, C. Momma, B. Wellegehausen, W. Becker, S. Long, J. K. McIver. Phys. Rev. A, 51, R3414(1995).
[19] L. V. Dao, S. Teichmann, J. Davis, P. Hannaford. J. Appl. Phys., 104, 023105(2008).
[20] T. Popmintchev, M. Chen, P. Arpin, M. M. Murnane, H. C. Kapteyn. Nat. Photonics, 4, 822(2010).
[21] P. Wei, J. Miao, Z. Zeng, C. Li, X. Ge, R. Li, Z. Xu. Phys. Rev. Lett., 110, 233903(2013).
[22] P. Wei, Z. Zeng, J. Jiang, J. Miao, Y. Zheng, X. Ge, C. Li, R. Li. Appl. Phys. Lett., 104, 151101(2014).
[23] P. Wei, Q. Tian, Z. Zeng, J. Jiang, J. Miao, Y. Zheng, X. Ge, C. Li, R. Li, Z. Xu. Laser Phys., 24, 085302(2014).
[24] G. Lambert, T. Hara, D. Garzella, T. Tanikawa, M. Labat, B. Carre, H. Kitamura, T. Shintake, M. Bougeard, S. Inoue, Y. Tanaka, P. Salieres, H. Merdji, O. Chubar, O. Gobert, K. Tahara, M.-E. Couprie. Nat. Phys., 4, 296(2008).
[25] M. Lewenstein, P. Salières, A. L’Huillier. Phys. Rev. A, 52, 4747(1995).
[26] P. Salières, B. Carré, L. Le Déroff, F. Grasbon, G. G. Paulus, H. Walther, R. Kopold, W. Becker, D. B. Milošević, A. Sanpera, M. Lewenstein. Science, 292, 902(2001).
[27] N. Milosevic, A. Scrinzi, T. Brabec. Phys. Rev. Lett., 88, 093905(2002).
[28] M. B. Gaarde, J. L. Tate, K. J. Schafer. J. Phys. B: At. Mol. Opt. Phys., 41, 132001(2008).
[29] P. Salières, A. L’Huillier, M. Lewenstein. Phys. Rev. Lett., 74, 3776(1995).