Liye Li, Yifan Ouyang, Lijun Ma, Hongshun Sun, Yusa Chen, Meizhang Wu, Zhimei Qi, Wengang Wu, "Reflection-type surface lattice resonances in all-metal metasurfaces for refractive index sensing," Photonics Res. 11, 2210 (2023)

Search by keywords or author
- Photonics Research
- Vol. 11, Issue 12, 2210 (2023)
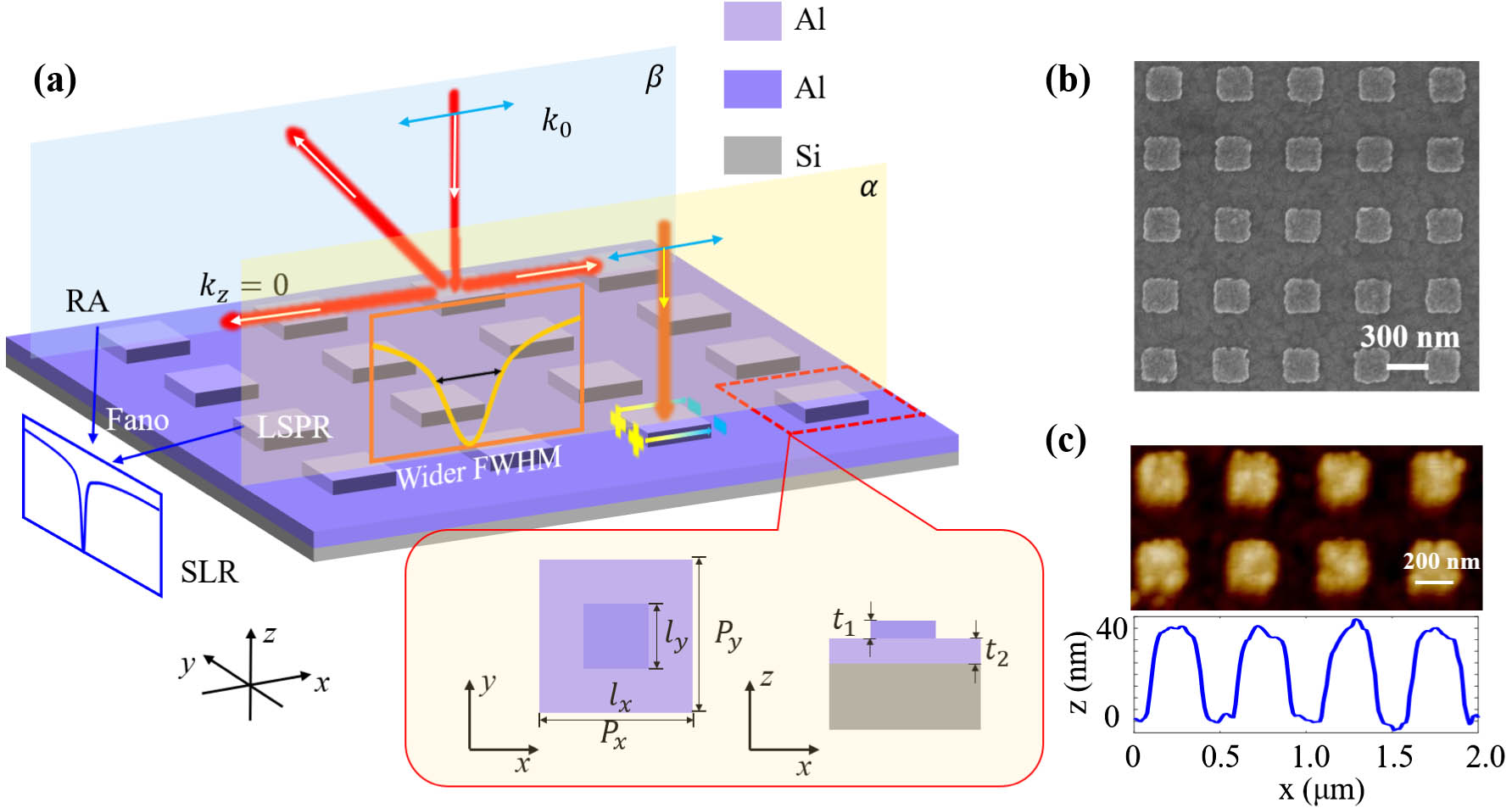
Fig. 1. Schematics and experimental characterization of the proposed all-metal metasurface. (a) Schematic illustration of the all-metal metasurface and the coupling between the LSPR and the RA. The structural parameters are as follows: P x = P y = 500 nm , l x = l y = 250 nm , t 1 = 40 nm , t 2 = 150 nm . (b) SEM image of the fabricated metasurface. (c) Smoothness and height characterizations of the metasurface sample by the AFM.

Fig. 2. Experimental and simulated results of the designed metasurface. (a) Measured and (b) numerical simulation reflection spectra of metasurfaces with different meta-atom widths of 200 nm, 250 nm, and 300 nm. Electric field E z distribution at the SLR wavelength (514.7 nm) in the (c) x – y plane and (d) x – z plane.
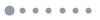
Fig. 3. RI sensing results of numerical simulation and measurement. (a) Variation of simulated SLR spectra with RI environments. (b) SLR dip positions in the spectra and corresponding calculated RI sensing sensitivity. (c) Measured reflection-type SLR spectra under air (n = 1.0000 ), deionized water (n = 1.3333 ), anhydrous alcohol (n = 1.3614 ), and isopropanol (n = 1.3772 ). (d) Measured SLR dip positions, RI sensitivity, and Q -factors. (e) Numerical simulation transmission-type SLR spectra under different RI environments. (f) SLR dip positions and RI sensitivities. The orange line is composed of four line segments with different gradients, with a low linearity. The inset is the designed transmission metasurface model and the length unit is in nanometers.
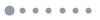
Fig. 4. Spectra and sensitivities of other metasurfaces. (a) Measured reflection-type SLR spectra in different RI environments and (b) RI sensitivity of the metasurface with P x = 600 nm and l x = 400 nm . The inset picture is the SEM image of the sample. Similarly, (c) and (d) are the reflectivity spectra, calculated RI sensitivity, and SEM image of the metasurface with P x = 700 nm and l x = 450 nm .
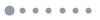
Fig. 5. Experimental results from angular multiplexing with different polarization modes. (a) Measured reflection spectra of the TM beam in the case of different incident angles from 10° to 50° at a 10° interval. (b) Measured reflection spectra of the TE beam with different incident angles from 5° to 25° at a 5° interval. Measured SLR spectra under the different RI conditions of the (c) TM beam and (d) TE beam ( θ i = 20 ° ) .
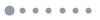
Fig. 6. Experimental results from a rectangular array. (a) SEM image of the all-metal metasurface with the rectangular array (P x = 500 nm , P y = 600 nm ) and rectangular meta-atom (l x = 250 nm , l y = 400 nm ). (b) Numerical simulation SLR spectra excited by orthogonally polarized incidences. (c), (d) Measured reflection spectra under different RI environments in the case of x - and y -polarizations, separately.
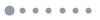
Fig. 7. Measured and simulated spectra and SEM images of metasurfaces with the (a) hexagonal meta-atom, (b) nanohole meta-atom, (c) hexagonal array, and (d) honeycomb array. The above SLR spectra are all excited by x -polarized incidence beams.
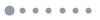
Fig. 8. (a) Theoretical calculation wavelengths of the k SPP and k RA . (b) Simulated spectra of the meta-atoms with widths of 150 nm and 100 nm.
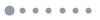
Fig. 9. (a) Simulated reflection spectra and (b) SLR dip positions of the metasurface with P x = 600 nm under different RI environments. (c) Simulated reflection spectra and (d) SLR dip positions of the metasurface with P x = 700 nm under different RI environments.
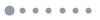
Fig. 10. (a), (b) Simulated reflection spectra and SLR dip positions of the Au metasurface under different RI environments. Inset image is the structure diagram with geometric parameters (P x = P y = 650 nm , l x = l y = 300 nm , t 1 = 40 nm ). (c), (d) Simulated reflection spectra and SLR wavelengths of the Ag metasurface, whose geometric parameters are as follows: P x = P y = 500 nm , l x = l y = 200 nm , t 1 = 40 nm .
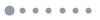
Fig. 11. Schematic diagrams of the (a) TM mode incidence and (b) TE mode incidence.
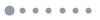
Fig. 12. Normalized electric field E z distributions on the meta-atom surface in the case of (a) x -polarization and (b) y -polarization.
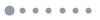
Fig. 13. (a) SEM image of the proposed metasurface with the rectangular array (P x = 500 nm and P y = 700 nm ) and rectangular meta-atom (l x = 250 nm , l y = 500 nm , and h = 50 nm ). (b), (c) Measured reflection spectra under different RI environments in the case of x - and y -polarizations, respectively. (d) SLR positions and corresponding RI sensitivities of both polarization states.
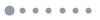
Fig. 14. Schematic diagrams of the (a) hexagonal array and (b) honeycomb array.
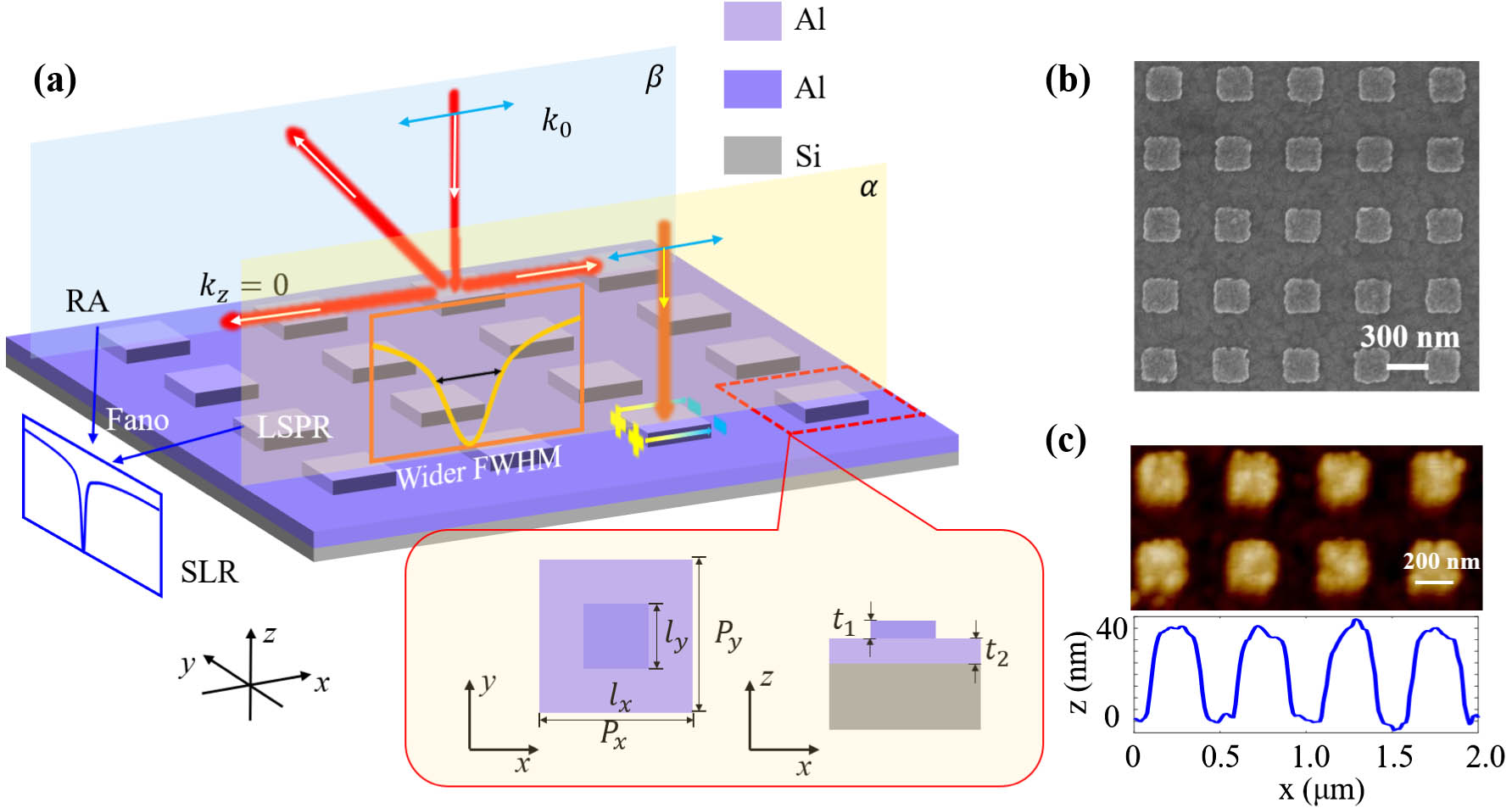
Set citation alerts for the article
Please enter your email address