Abstract
In this paper, we propose and experimentally investigate a linearly polarized narrow-linewidth random fiber laser (RFL) operating at 1080 nm and boost the output power to kilowatt level with near-diffraction-limited beam quality using a master oscillation power amplifier. The RFL based on a half-opened cavity, which is composed of a linearly polarized narrow-linewidth fiber Bragg grating and a 500 m piece of polarization-maintained Ge-doped fiber, generates a 0.71 W seed laser with an 88 pm full width at half-maximum (FWHM) linewidth and a 22.5 dB polarization extinction ratio (PER) for power scaling. A two-stage fiber amplifier enhances the seed laser to the maximal 1.01 kW with a PER value of 17 dB and a beam quality of and . No stimulated Brillouin scattering effect is observed at the ultimate power level, and the FWHM linewidth of the amplified random laser broadens linearly as a function of the output power with a coefficient of about . To the best of our knowledge, this is the first demonstration of a linearly polarized narrow-linewidth RFL with even kilowatt-level near-diffraction-limited output, and further performance scaling is ongoing.1. INTRODUCTION
Random fiber lasers (RFLs) based on the extremely weak Rayleigh scattering in a piece of passive fiber that provide random distributed feedback (RDFB) have found various applications, such as sensing technology and spectroscopic monitoring, due to the fact that they are mode-free and mirror-free and feature low coherence length and structural simplicity [1–3]. In recent years, many investigations of RFLs have been presented on their power enhancing [4–7] and performance scaling in the temporal, spatial, and spectral domains [8–15]. Furthermore, explorations of the application of RFLs have also taken place [16–19].
Narrow-linewidth oscillation of an RFL, as a good candidate for investigating temporal and statistical properties and the necessary requirements for many applications, have been widely attempted by spectral filtering with the aid of a fiber Bragg grating (FBG), Fabry–Perot filter, high-finesse narrowband Fabry–Perot interferometer, and so on [17,20–25]. It’s worth noting that the highest output power from a narrow-linewidth RFL up to now was limited to 2.15 W with about a 0.16 nm full width at half-maximum (FWHM) linewidth, which was randomly polarized [25]. As previous works have proven that a narrow-linewidth incoherent seed, e.g., a superfluorescent fiber source, can suppress the stimulated Brillouin scattering (SBS) generation in a high-power narrow-linewidth fiber source with at least 17 dB, resulting from unique mode-free and low-coherence-length features [26,27], the investigation of power scaling of a narrow-linewidth RFL is significant. At the same time, the linearly polarized emissions from RFLs have also been widely studied, with output power enhancement from the watt level to the 100 W level and a typical FWHM linewidth of several nanometers [28–32]. At this time, despite the demonstration of a watt-level randomly polarized narrow-linewidth RFL [25] and a 100-watt-level linearly polarized RFL [32] in a single-stage scheme, investigations of linearly polarized narrow-linewidth RFLs have not been reported, and further power amplification is also worth attempting.
In this contribution, we propose and experimentally explore a linearly polarized narrow-linewidth RFL and scale the random laser to the kilowatt level with a 212 pm FWHM linewidth and near-diffraction-limited beam quality via an all-fiberized master oscillation power amplifier (MOPA) scheme. Random laser seed with a maximal output power 0.71 W can be obtained from the linearly polarized narrow-linewidth RFL with a FWHM linewidth of 88 pm and polarization extinction ratio (PER) value of 22.5 dB. As for the main scaling stage, a 1.01 kW amplified random laser can be achieved with a 212 pm FWHM linewidth and near-diffraction-limited beam quality. The linear evolution of the output power and monitored backward power indicates that no SBS effect is generated in the power scaling process and further power scaling is available with a more powerful pump source.
Sign up for Photonics Research TOC. Get the latest issue of Photonics Research delivered right to you!Sign up now
2. EXPERIMENTAL SETUP
Figure 1 plots the schematic of the demonstrated high-power linearly polarized narrow-linewidth RFL in MOPA structure. To obtain a high-power random laser, a two-stage amplification chain is utilized after the linearly polarized narrow linewidth seed laser with relatively low-level power. The seed laser employs a half-opened cavity, which is composed of a polarization-maintained (PM) FBG centered at 1080 nm with high reflectivity (99%) and narrow reflection linewidth (80 pm) and a piece of 500 m long PM Ge-doped fiber (GDF) with a core/inner cladding diameter of 10/125 μm and numerical aperture (NA) of 0.07. A half-opened cavity can be achieved with the point feedback (PFB) provided by the FBG and RDFB offered by the passive fiber. The gain of the random laser is generated from Raman gain in the passive fiber. Narrowband oscillation of the RFL can be obtained with the aid of a narrow-linewidth FBG via the selection of initial broadband spontaneous Raman emission in the passive fiber. The sections of the initial spontaneous Raman emission out of the reflection band and the residual pump laser are stripped from the 8° angle cleaved (to suppress the Fresnel reflection) end of the FBG. The pump laser centered at 1041 nm from a linearly polarized fiber laser is injected into the half-opened cavity via the first port of a fiber circulator (CIR 1), and the narrow-linewidth linearly polarized random laser is coupled out from the cavity with the aid of the third port of CIR 1. To purify the spectrum of the seed laser from the RFL, a bandpass filter (BPF) with a operation region is spliced with the third port of CIR 1.
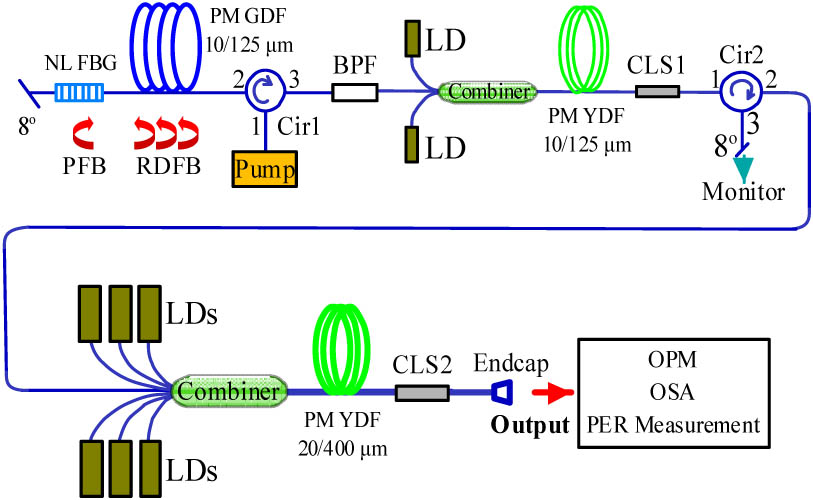
Figure 1.Schematic of the kilowatt-level linearly polarized narrow-linewidth RFL. NL, narrow linewidth; Cir, circulator; OPM, optical power meter; OSA, optical spectrum analyzer.
To scale the seed power to a suitable level, a preamplifier is inserted between the narrow-linewidth seed laser and the main amplifier, which consists of a combiner, two 20-W-level laser diodes (LDs), a 5 m long piece of PM dual-cladding Yb-doped fiber (YDF; average cladding absorption coefficient for 976 nm pump light), and a cladding light stripper (CLS 1). To strip the backward light and protect the components of the preamplifier and seed laser, a fiber circulator (CIR 2), which is the same type as CIR 1, is utilized after CLS 1. The pump delivery fibers of the LDs and combiner are multimode fiber with 105/125 μm and 0.22 NA. The core/inner cladding diameter and NA of the pigtailed fiber of the signal port of combiner, CLS 1, and CIR 2 are 10/125 μm and 0.07, respectively. The main power amplification stage is a dual-cladding YDF amplifier. The preamplified seed laser and pump light from six 240-W-level LDs are launched into the PM gain fiber via the combiner, whose input and output signal ports exhibit 10/125 μm and 20/400 μm core/inner cladding diameters, respectively. The pump delivery fibers of the LDs and combiner are multimode fibers with 220/242 μm and 0.22 NA. The gain fiber employs a segment of 10 m long PM YDF exhibiting a 20/400 μm core/inner cladding diameter with a 0.06/0.46 NA, and the average cladding absorption coefficient for 976 nm pump light is about . For the stripping of possible cladding light in the PM YDF, CLS 2 is spliced with the gain fiber. An endcap coated with antireflection films is utilized to deliver the amplified random laser into free space more safely. The PM YDF is coiled on the surface of a water-cooling aluminum plate with a diameter of about 13 cm, and the temperature of the cooling water is controlled to be 20°C with the aid of a laser cooler.
3. EXPERIMENTAL RESULTS AND DISCUSSION
The output characteristics of the linearly polarized narrow-linewidth random laser from the third port of CIR 1 are displayed in Fig. 2. The pump threshold for random laser oscillation is about 6.0 W, as plotted in Fig. 2(a). With the scaling of the pump power from 6.0 W to pump-source-limited 25.1 W, the output power grows rapidly from 0.01 to 0.71 W. Furthermore, the slight spectral broadening of the output random laser can be observed in the power-enhancing process and the FWHM linewidth broadens from an initial to a stable value of . Generally speaking, the FWHM linewidth is maintained well () in the power boosting process, which may benefit from the relatively low operating power level of the random laser and the short length of the utilized passive fiber, as the spectral broadening is mainly induced by effects such as self-phase modulation (SPM) and cross-phase modulation (XPM) [30]. The output spectrum at the maximum power level is measured by an optical spectrum analyzer (OSA) with a 0.02 nm optical resolution and shown in Fig. 2(b). The optical signal-to-noise ratio of the output spectrum is about 32.5 dB and is limited by the optical peak corresponding to the residual pump laser, which may be induced by the cross talk between the ports of the fiber circulator and the distributed reflection of the pump light by the passive fiber, as the passive fiber can act as distributed mirror [33]. Furthermore, the broad optical pedestal covering a 1060–1100 nm range, as depicted in the inset of Fig. 2(b), may be caused by the backward spontaneous Raman emission of the pump laser and the distributed reflection of the forward spontaneous Raman emission [21]. The polarization characteristics of the employed pump laser and output random laser are measured by spatial PER measurement, which is the same as that utilized in Ref. [31], and presented in Fig. 2(c). The PER value of the random laser can reach as high as 22.5 dB at maximum output power, and the PER values of the random laser are slightly higher than those of the pump laser, which may benefit from the polarization-sensitive feature of the Raman gain process [34]. With the aid of the BPF, the residual pump laser in the output light can be prevented. However, the spontaneous Raman component in the operation range of the BPF can still be transmitted, and the linearly polarized narrow-linewidth seed is preamplified to 20.0 W with an FWHM linewidth of 90 pm before the power boosting of the main amplifier.
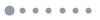
Figure 2.Output characteristics of the linearly polarized narrow-linewidth seed. (a) Output power and FWHM linewidth as functions of pump power, (b) output spectrum at the maximum power level, (c) evolution of the PERs of the pump laser and random laser as functions of pump power.
Figure 3 plots the output characteristics of the main amplification stage. For a given 1.38 kW pump light and 20.0 W preamplified seed laser, the amplifier obtains an ultimate output power of 1.01 kW, corresponding to an optical-to-optical conversion efficiency of 71.74%. Furthermore, the backward power increases almost linearly with the enhancing of the pump power, which indicates that no SBS effect is generated in the power scaling process. The spectral characteristic of the amplified random laser at maximum output power is depicted in Fig. 3(b). Most of the 976 nm pump light can be absorbed or stripped, and the residual 976 nm pump light is 41.4 dB lower than the amplified random laser. Additionally, the optical peak corresponding to the Raman gain of the 1080 nm random laser can be measured, with an amplitude about 25 dB lower than the amplified random laser. Using numerical integrating, 93.7% of the output power is within the confined spectral window (1077–1083 nm). A beam quality of and is measured using the method by an Ophir-Spiricon beam propagation analyzer, as displayed in Fig. 3(c). Furthermore, the polarization characteristic of random laser is maintained well in the power amplification process, with around a 17 dB level. In contrast with the seed laser, the slight decreasing of the polarization feature may be caused by the transmission of the amplifier and the power amplification process. As the output power increases linearly with the increment of the pump power and no power rollover can be observed in the power scaling process, we believe that further power scaling is available with a more powerful pump source.
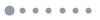
Figure 3.Characteristics of the main amplifier. (a) Output and backward power as functions of pump power, (b) output spectrum at maximum power, (c) measurement of beam quality at full power.
As the narrow linewidth feature is very important for many applications, such as beam combination, the evolution of the output spectrum of this powerful narrow linewidth random laser is investigated in detail and presented in Fig. 4. With the enhancement of the output power to 1.01 kW, the FWHM linewidth broadens from 90 to 212 pm. The linear fitting curve of the FWHM linewidth as a function of output power indicates that the output spectrum broadens gradually with a coefficient of about , which is different from the previously presented kilowatt-level spectral-broadening-free amplifier in Ref. [6] seeded by a randomly polarized broad-linewidth (about 1 nm) random laser. As the spectral broadening is mainly induced by the generation of nonlinear effects [35], such as SPM and XPM, the difference in the spectral broadening may be caused by the variation of the initial FWHM linewidth and polarization state of the injected seed, fiber length of the amplifier, and so on. Significantly, the spectral broadening coefficient is smaller than that in the reported kilowatt-level all-fiberized system for spectral beam combination presented in Ref. [27], seeded by an incoherent narrow-linewidth superfluorescent fiber source (about ). This proves that employing an incoherent narrow-linewidth random laser seed to obtain a powerful narrowband laser system also has an advantage in maintaining linewidth, besides the superiority in SBS suppression.
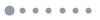
Figure 4.Spectral broadening of the amplified random laser.
To the best of our knowledge, this is the first investigation of a linearly polarized narrow-linewidth RFL with even kilowatt-level near-diffraction-limited output. Compared with our previously reported randomly polarized narrow-linewidth RFL in Ref. [25], whose minimum FWHM linewidth of the output laser was limited to , the optimization of the seed laser is attempted in this presentation via the passive fiber length shortening to obtain a narrow-linewidth seed with a FWHM linewidth of for successive power scaling. Simultaneously, the linearly polarized operation of a narrow-linewidth RFL is realized with a PER value as high as 22.5 dB via the improved performance due to the pump source and fiber devices. Furthermore, unlike the kilowatt-level randomly polarized broad-linewidth RFL system with its spectral-broadening-free property in Ref. [6], the linear broadening of the FWHM linewidth can be measured via the enhancement of output power. Hence, the influences of the initial linewidth, polarization state, and parameters of the power amplifier on the output spectrum evolution are worth investigating further.
4. SUMMARY
In conclusion, we have proposed a linearly polarized narrow-linewidth RFL and boosted the output power to the kilowatt level with near-diffraction-limited beam quality by employing an all-fiber MOPA scheme. The linearly polarized narrow-linewidth RFL operating at 1080 nm in a half-opened cavity pumped by a linearly polarized fiber laser employs a narrow-linewidth FBG to obtain narrowband oscillation. The corresponding linewidth and PER of the random laser seed at a maximal 0.71 W are about 88 pm and 22.5 dB, respectively. As for the main amplifier, the maximum output power of 1.01 kW can be achieved with an optical-to-optical conversion efficiency of 71.74%, and 93.7% of the total output power remains within the steep center peak. A near-diffraction-limited ( and ) amplified random laser can be achieved with a PER value of about 17 dB. The FWHM linewidth broadens gradually to 212 pm at the ultimate output power with a coefficient of , indicating that the employment of a narrow-linewidth random laser as the seed of the powerful narrowband fiber source is comparatively competitive. The linear increment tendency of the output power and monitored backward power as functions of the pump power indicates that no SBS effect is generated in the power scaling process and further power scaling is available with a more powerful pump source. The demonstration provides a potential powerful linearly polarized narrow-linewidth fiber source for many applications, and further investigation of the influence of the operating parameters on the spectral evolution and the performance scaling of this system is ongoing.
Acknowledgment
Acknowledgment. We are particularly grateful to Xiaolin Wang, Rongtao Su, and Sen Guo for their support of this work.
References
[1] D. S. Wiersma. The physics and applications of random lasers. Nat. Phys., 4, 359-367(2008).
[2] S. K. Turitsyn, S. A. Babin, A. E. El-Taher, P. Harper, D. V. Churkin, S. I. Kablukov, J. D. Ania-Castañón, V. Karalekas, E. V. Podivilov. Random distributed feedback fibre laser. Nat. Photonics, 4, 231-235(2010).
[3] X. Du, H. Zhang, H. Xiao, P. Ma, X. Wang, P. Zhou, Z. Liu. High-power random distributed feedback fiber laser: from science to application. Ann. Phys., 528, 649-662(2016).
[4] H. Zhang, P. Zhou, H. Xiao, X. Xu. Efficient Raman fiber laser based on random Rayleigh distributed feedback with record high power. Laser Phys. Lett., 11, 075104(2014).
[5] Z. Wang, H. Wu, M. Fan, L. Zhang, Y. Rao, W. Zhang, X. Jia. High power random fiber laser with short cavity length: theoretical and experimental investigations. IEEE J. Sel. Top. Quantum Electron., 21, 0900506(2015).
[6] X. Du, H. Zhang, P. Ma, H. Xiao, X. Wang, P. Zhou, Z. Liu. Kilowatt-level fiber amplifier with spectral broadening-free property, seeded by a random fiber laser. Opt. Lett., 40, 5311-5314(2015).
[7] X. Du, H. Zhang, X. Wang, P. Zhou, Z. Liu. Short cavity-length random fiber laser with record power and ultrahigh efficiency. Opt. Lett., 41, 571-574(2016).
[8] Y. Tang, J. Xu. A random Q-switched fiber laser. Sci. Rep., 5, 9338(2015).
[9] B. Yao, Y. Rao, Z. Wang, Y. Wu, J. Zhou, H. Wu, M. Fan, X. Cao, W. Zhang, Y. Chen, Y. Li, D. Churkin, S. Turitsyn, C. W. Wong. Graphene based widely-tunable and singly-polarized pulse generation with random fiber lasers. Sci. Rep., 5, 18526(2015).
[10] H. Wu, Z. Wang, Q. He, M. Fan, Y. Li, W. Sun, L. Zhang, Y. Li, Y. Rao. Polarization-modulated random fiber laser. Laser Phys. Lett., 13, 055101(2016).
[11] X. Du, H. Zhang, P. Ma, X. Wang, P. Zhou, Z. Liu. Spatial mode switchable fiber laser based on FM-FBG and random distributed feedback. Laser Phys., 25, 095102(2015).
[12] I. A. Lobach, S. I. Kablukov, M. I. Skvortsov, E. V. Podivilov, M. A. Melkumov, S. A. Babin, E. M. Dianov. Narrowband random lasing in a Bismuth-doped active fiber. Sci. Rep., 6, 30083(2016).
[13] X. Jin, Z. Lou, H. Zhang, J. Xu, P. Zhou, Z. Liu. Random distributed feedback fiber laser at 2.1 μm. Opt. Lett., 41, 4923-4926(2016).
[14] I. D. Vatnik, E. A. Zlobina, S. I. Kablukov, S. A. Babin. Multi-peak structure of generation spectrum of random distributed feedback fiber Raman lasers. Opt. Express, 25, 2703-2708(2017).
[15] L. Zhang, H. Jiang, X. Yang, W. Pan, S. Cui, Y. Feng. Nearly-octave wavelength tuning of a continuous wave fiber laser. Sci. Rep., 7, 42611(2017).
[16] H. Zhang, P. Zhou, X. Wang, X. Du, H. Xiao, X. Xu. Hundred-watt-level high power random distributed feedback Raman fiber laser at 1150 nm and its application in mid-infrared laser generation. Opt. Express, 23, 17138-17144(2015).
[17] E. I. Dontsova, S. I. Kablukov, I. D. Vatnik, S. A. Babin. Frequency doubling of Raman fiber lasers with random distributed feedback. Opt. Lett., 41, 1439-1442(2016).
[18] P. Rosa, G. Rizzelli, M. Tan, P. Harper, J. D. Ania-Castañón. Characterisation of random DFB Raman laser amplifier for WDM transmission. Opt. Express, 23, 28634-28639(2015).
[19] M. Tan, P. Rosa, S. T. Le, M. A. Iqbal, I. D. Phillips, P. Harper. Transmission performance improvement using random DFB laser based Raman amplification and bidirectional second-order pumping. Opt. Express, 24, 2215-2221(2016).
[20] S. Sugavanam, N. Tarasov, X. Shu, D. V. Churkin. Narrow-band generation in random distributed feedback fiber laser. Opt. Express, 21, 16466-16472(2013).
[21] J. Xu, J. Ye, H. Xiao, J. Leng, J. Wu, H. Zhang, P. Zhou. Narrow-linewidth Q-switched random distributed feedback fiber laser. Opt. Express, 24, 19203-19210(2016).
[22] M. Pang, X. Bao, L. Chen. Observation of narrow linewidth spikes in the coherent Brillouin random fiber laser. Opt. Lett., 38, 1866-1868(2013).
[23] D. Leandro, S. Rota-Rodrigo, D. Ardanaz, M. Lopez-Amo. Narrow-linewidth multi-wavelength random distributed feedback laser. J. Lightwave Technol., 33, 3591-3596(2015).
[24] T. Zhu, F. Chen, S. Huang, X. Bao. An ultra-narrow linewidth fiber laser based on Rayleigh backscattering in a tapered optical fiber. Laser Phys. Lett., 10, 055110(2013).
[25] J. Ye, J. Xu, H. Zhang, P. Zhou. Powerful narrow linewidth random fiber laser. Photon. Sens., 7, 82-87(2017).
[26] O. Schmidt, M. Rekas, C. Wirth, J. Rothhardt, S. Rhein, A. Kliner, M. Strecker, T. Schreiber, J. Limpert, R. Eberhardt, A. Tünnermann. High power narrow-band fiber-based ASE source. Opt. Express, 19, 4421-4427(2011).
[27] Y. Zheng, Y. Yang, J. Wang, M. Hu, G. Liu, X. Zhao, X. Chen, K. Liu, C. Zhao, B. He, J. Zhou. 10.8 kW spectral beam combination of eight all-fiber superfluorescent sources and their dispersion compensation. Opt. Express, 24, 12063-12071(2016).
[28] X. Du, H. Zhang, X. Wang, P. Zhou, Z. Liu. Investigation on random distributed feedback Raman fiber laser with linear polarized output. Photon. Res., 3, 28-31(2015).
[29] Z. Hu, Y. Liang, X. Qian, P. Xie, H. Jiang. Polarized random laser emission from an oriented disorder polymer optical fiber. Opt. Lett., 41, 2584-2587(2016).
[30] S. A. Babin, E. A. Zlobina, S. I. Kablukov, E. V. Podivilov. High-order random Raman lasing in a PM fiber with ultimate efficiency and narrow bandwidth. Sci. Rep., 6, 22625(2016).
[31] J. Xu, P. Zhou, J. Leng, J. Wu, H. Zhang. Powerful linearly-polarized high-order random fiber laser pumped by broadband amplified spontaneous emission source. Sci. Rep., 6, 35213(2016).
[32] J. Xu, Z. Lou, J. Ye, J. Wu, J. Leng, H. Xiao, H. Zhang, P. Zhou. Incoherently pumped high-power linearly-polarized single-mode random fiber laser: experimental investigations and theoretical prospects. Opt. Express, 25, 5609-5617(2017).
[33] A. M. R. Pinto, M. Lopez-Amo, J. Kobelke, K. Schuster. Temperature fiber laser sensor based on a hybrid cavity and a random mirror. J. Lightwave Technol., 30, 1168-1172(2012).
[34] G. P. Agrawal. Nonlinear Fiber Optics(2013).
[35] W. Liu, H. Xiao, X. Wang, P. Zhou. Study on output spectral characteristic of Yb-doped fiber lasers. Chin. J. Lasers, 40, 0902006(2013).