Abstract
We report a compact Mach–Zehnder interferometer (MZI) electro-optic switch fabricated on a silicon-on-insulator using standard complementary metal-oxide semiconductor (CMOS) processes. With a short modulation arm length of 200 μm, the crosstalk is reduced to by the new modulation scheme of push–pull modulation with a pre-biased phase shift. The new modulation scheme can also work with a fast switching time of about 5.4 ns.Silicon photonics, with their high capacity and compatibility with standard complementary metal-oxide semiconductor (CMOS) processes, have been attracting increasing attention[1–6]. High-speed optical switches fabricated on silicon-on-insulators (SOIs) offer a promising approach to eliminate the bottlenecks that occur with conventional electronic switches. Optical switches based on symmetrical Mach–Zehnder interferometer (MZI) structures, which operate on a broad wavelength range, have been widely studied[7–14]. Usually, the electro-optic switches based on the free-carrier dispersion (FCD) effect[7] have a fast switching time on a nanosecond scale[8], which is much faster than the sub-microsecond-scale switching time of thermal-optic switches[11]. However, during the electro-optic phase modulation, significant optical loss will also be caused by the free-carrier absorption (FCA)[7–9], which is a big disadvantage compared with the thermal-optic effect. When the electro-optic phase modulation is applied to a single modulation arm of the MZI, which can be called the single-arm modulation scheme, the original balance of the optical powers between the two modulation arms will be destroyed. Another problem is the self-heating effect caused by the current during the electro-optic modulation, which unfortunately goes against the FCD effect[8]. When large power consumption is required to obtain the phase shift, an obvious self-heating effect will be induced, and the imbalance of the optical powers will become more serious. As a result of imbalanced interference, using the single-arm modulation scheme, it is difficult to reduce the crosstalk (CT) of a MZI-based electro-optic switch to less than . In Ref. [10], when the lengths of the modulation arms are as short as 200 μm, the CT is as large as . And in Ref. [8], after reducing the self-heating effect with 4 mm-long modulation arms, the CT only decreases to . Meanwhile, without the disadvantages of FCA, the CT of MZI-based thermal-optic switches can be easily reduced to when fabricated on a SOI[11,12]. In our previous work[13], using a double-gate configuration, an ultra-low CT of was reported. However, a much more complex and larger footprint is required, which is not suitable for large-scale integration.
To solve the CT problems of a MZI-based electro-optic switch under the single-arm modulation scheme, different modulation schemes have also been reported. One is the double-arm modulation with a pre-biased phase shift[7], which has realized a CT of with modulation arms longer than 1 mm. The other is the push–pull modulation[9], which introduces medium-level background doping into the 2.5 mm-long modulation arms and can give a low CT of in simulations.
In this Letter, we report a SOI-based MZI electro-optic switch with a low CT and a compact footprint. The switch works under a novel modulation scheme, which can be called push–pull modulation with a pre-biased phase shift. The novel scheme combines the advantages of the double-arm modulation and the push–pull modulation, which is realized by introducing medium-level background doping and a phase shifter into the modulation arms. Under the push–pull modulation with a pre-biased phase shift, a low CT of can be obtained with 200 μm-long modulation arms.
Sign up for Chinese Optics Letters TOC. Get the latest issue of Chinese Optics Letters delivered right to you!Sign up now
The schematic diagram of a MZI-based electro-optic switch is shown in Fig. 1(a). It includes a pair of 3 dB splitters (combiners) and two symmetrical phase shifters (modulation arms). To make the switch both broadband and compact, multimode interference (MMI) couplers are chosen as the 3 dB splitters[8]. The phase mismatch is defined as the difference between the two phase shifters (). When the phase mismatch is 0 or , the switch is in the “off” state or the “on” state, respectively. Electro-optic modulation can be realized by applying either free-carrier injection under forward bias (represented by “+Arm1” and “+Arm2”) or free-carrier depletion under reverse bias (represented by “−Arm1” and “−Arm2”) to the two arms, which will result in a decreased or increased modulation of the effective index, respectively[9]. As shown in Fig. 1(b), when the two junctions were reversely connected in parallel, forward injection and reverse depletion can be realized simultaneously, which is called the push–pull configuration. The forward injection is more efficient than the reverse depletion when the applied voltages have the same absolute values.
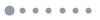
Figure 1.(a) Schematic diagram of a MZI-based electro-optic switch. (b) Equivalent circuit of the switch with a push–pull configuration.
A switch, which is suitable for large-scale photonics switching networks, should have a low CT and a compact footprint at the same time. The schematic diagram of the single-arm modulation scheme is shown in Fig. 2(a). When using short modulation arms from 200 to 400 μm in length[13,14], a large power of about 10 mW is required to realize the phase shift of on a single arm. As a result of the large power, a significant self-heating effect, which acts in the opposite direction of free-carrier-induced index change, will make the required amplitude of the electro-optic phase modulation larger[8]. The loss caused by FCA is correlated positively with the amplitude of the electro-optic phase modulation. The two reported modulation schemes, the push–pull modulation and the double-arm modulation with a pre-biased phase shift, are shown in Figs. 2(b) and 2(c), respectively. In the push–pull modulation, positive and negative voltages with the same absolute value are simultaneously applied to “Arm1” and “Arm2,” respectively. The total modulation effects of the two arms enlarge the phase mismatch from 0 to , making the phase shift of each arm smaller than . The phase shift of “Arm1” is much larger than that of “Arm2,” because forward injection is more efficient than the reverse depletion. In the double-arm modulation with a pre-biased phase shift, the switch is originally designed with a phase mismatch, which can be called the “original” state. The phase mismatch can be achieved by adding an additional waveguide or by changing the width of waveguide. The switch from the “original” state to the “on” or the “off” state is realized by applying positive voltage to “Arm1” or “Arm2,” separately. Thus, the phase shift on each arm is . What can be clearly seen is that both of the reported modulation schemes focus on reducing the amplitude of the phase modulation on a single arm by distributing the total phase shift of over two arms[7,9]. Without taking the self-heating effect into account, the amplitude of the electro-optic phase modulation on a single arm has been reduced to by the scheme of the double-arm modulation with a pre-biased phase shift.
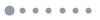
Figure 2.Schematic diagrams of different modulation schemes. (a) Single-arm modulation. (b) Push–pull modulation. (c) Double-arm modulation with a pre-biased phase shift. (d) Push–pull modulation with a pre-biased phase shift.
The amplitude of the phase modulation on a single arm can be further reduced to less than with a better modulation scheme, which combines the advantages of the two reported schemes together. This new modulation scheme can be called push–pull modulation with a pre-biased phase shift[15], as shown in Fig. 2(d). To explain the advantages of the new modulation scheme, the phase mismatch modulation from to (from the “original” state to the “on” state) is taken as an example. Positive and negative voltages with the same absolute value are simultaneously applied to “Arm1” and “Arm2,” respectively. The total modulation effects of the two arms enlarge the phase mismatch from to , making the phase shift of each arm smaller than . The phase shift of “Arm1” is much larger than that of “Arm2,” because forward injection is more efficient than the reverse depletion. Without taking the self-heating effect into consideration, the total amplitude of the phase modulation of this new modulation scheme over the two arms is also , which is the same as the double-arm modulation with a pre-biased phase shift[7]. The imbalance of the optical powers induced by the phase modulation is also the same. However, considering the self-heating effect, this new modulation scheme will have a small self-heating effect for the forward-injection arm and no self-heating effect for the reverse-depletion arm. All of these properties will make this scheme better than the double-arm modulation with a pre-biased phase shift in reducing the amplitude of the electro-optic phase modulation. This new modulation scheme will also be adopted in our MZI-based electro-optic switch to realize a low CT with short modulation arms.
The MZI-based electro-optic switch was fabricated on a SOI wafer by 0.18 μm standard CMOS processes from the Semiconductor Manufacturing International Corporation (SMIC) in China. The top silicon film layer is 340 nm, and the buried layer is 2 μm. As shown in Fig. 3(a), fiber-grating couplers with about a 3 dB coupling loss at the central wavelength were used for input/output (I/O) coupling at the four ports. Both modulation arms contain a 200 μm-long active diode, with a constant phase mismatch of between the two arms. Figure 3(b) shows the doping profile of the waveguide used as a modulation arm. A medium-level N-type background doping density of was used in the waveguide core region. Both the and the regions have a high doping density of to form good junctions. The gap between the rib edges and the () regions was optimized to 400 nm. More highly doped regions farther away from the rib edges were used to form good ohmic contact with the 1 μm-thick aluminum ground–signal–ground electrodes.
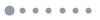
Figure 3.(a) The fabricated SOI-based electro-optic switch. (b) The doping profile of the waveguide used as a modulation arm.
To form the push–pull configuration shown in Fig. 1(b), where the two junctions were reversely connected in parallel, the electrodes were linked with gold wires [see Fig. 3(a)]. In this way, the new modulation scheme of push–pull modulation with a pre-biased phase shift is finally achieved. As shown in Fig. 2(d), when the electrical drive is negative, Arm1 works in the depletion mode (−Arm1), while Arm2 works in the injection mode (+Arm2). The effective index of Arm1 increases, while the effective index of Arm2 decreases. Then, the phase mismatch decreases from to 0, leading to an “off” state. When the electrical drive is positive, it will work in the opposite way, leading to the “on” state.
Figure 4 shows the measured optical power transmission from ports and when light was inputted from port 1, which was taken as an example to study the switching performance of the fabricated switch. Without the electrical drive, the difference in the optical power between the two output ports was very small (less than 3 dB), which was in agreement with the pre-biased phase shift. Under a negative electrical drive of (, 3.88 mW), the switch was modulated to the “off” state. Under a positive electrical drive of 1.01 V (7 mA, 7.07 mW), the switch was modulated to the “on” state. Thus, the push–pull modulation with a pre-biased phase shift is successfully experimentally achieved. With this new modulation scheme, the CTs of the “off” and “on” states were also largely reduced to and , respectively. The small difference between the two states was caused by fabrication errors and can be reduced by improving the fabrication process[10]. Considering that the lengths of the modulation arms are only 200 μm, the MZI-based electro-optic switch with both a low CT and a compact footprint is realized.
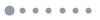
Figure 4.Normalized transmission as a function of the applied current under the push–pull modulation with a pre-biased phase shift at a wavelength of 1530 nm.
The transmission spectra of all optical paths at both switching states were measured over a large wavelength range from 1460 to 1600 nm. To remove the influence of the grating couplers, the spectra were normalized by a reference waveguide fabricated near the switch. As shown in Fig. 5, the switch performs broadband operations. In the 20 nm wavelength range around 1530 nm, the CT value remains below . The measured on-chip insertion loss (without the coupling loss) was about 3 dB around 1530 nm for both states, which can be attributed to the I/O tapers (about 0.2 dB), doping absorption (about 0.2 dB), phase modulation (about 0.4 dB), waveguide propagation (about 0.6 dB), and MMI splitters (about 1.6 dB).
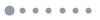
Figure 5.Measured transmission spectra under the push–pull modulation with a pre-biased phase shift.
To study the switching speed of the push–pull modulation with a pre-biased phase shift, a 50 MHz square-wave voltage signal with rising and falling times of about 170 ps was used as the electrical drive. As shown in Fig. 6, the 10%–90% rising (falling) time and falling (rising) time were 5.32 and 5.41 ns for the transmission of (), respectively. This nanosecond-scale switching time corresponds to the free-carrier injection process (whether a positive or negative electrical drive is applied), which will always exist in this kind of push–pull modulation scheme.
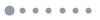
Figure 6.Measured switching time of the electro-optic switch under the push–pull modulation with a pre-biased phase shift.
In conclusion, a compact SOI-based MZI electro-optic switch with a low CT is proposed and experimentally demonstrated. To reduce the amplitude of the electro-optic phase modulation on a single arm to less than , a new modulation scheme of push–pull modulation with a pre-biased phase shift is introduced into a MZI-based switch. With 200 μm-long modulation arms, the CT value is reduced from [10] to less than . The fabrication processes are compatible with low-cost, standard CMOS processes. With a low CT, compact footprint, and nanosecond-scale fast switching time, the SOI-based MZI electro-optic switch should have a promising future in high-speed photonics switching networks.
References
[1] Z. Zhou, Z. Tu, B. Yin, W. Tan, L. Yu, H. Yi, X. Wang. Chin. Opt. Lett., 11, 012501(2013).
[2] Y. A. Vlasov. IEEE Commun. Mag., 50, S67(2012).
[3] J. Yang, S. Xu, K. Zhou, J. Xu. Chin. Opt. Lett., 12, S10501(2014).
[4] J. Liao, M. Marko, H. Jia, W. Sun, J. Liu, Y. Tan, J. Yang, X. Wang, Y. Zhang, W. Tang, X. Li, C. W. Wong. Chin. Opt. Lett., 12, S11905(2014).
[5] J. Y. Wu, P. Cao, T. Pan, Y. X. Yang, C. Y. Qiu, C. Tremblay, Y. K. Su. Photon. Res., 3, 9(2015).
[6] L. J. Lu, L. J. Zhou, X. W. Li, J. P. Chen. Opt. Lett., 39, 1633(2014).
[7] H. F. Zhou, Y. Zhao, W. J. Wang, J. Y. Yang, M. H. Wang, X. Q. Jiang. Opt. Express, 17, 7043(2009).
[8] P. Dong, S. Liao, H. Liang, R. Shafiiha, D. Feng, G. Li, X. Zheng, A. V. Krishnamoorthy, M. Asghari. Opt. Express, 18, 25225(2010).
[9] D. G. Sun, Z. M. Hu, S. Abdul-Majid, R. Vandusen, Q. Zheng, I. Hasan, N. G. Tarr, S. Bidnyk, T. J. Hall. J. Lightwave Technol., 29, 2592(2011).
[10] M. Yang, W. M. J. Green, S. Assefa, J. Van Campenhout, B. G. Lee, C. V. Jahnes, F. E. Doany, C. L. Schow, J. A. Kash, Y. A. Vlasov. Opt. Express, 19, 47(2011).
[11] L. Chen, Y. K. Chen. Opt. Express, 20, 18977(2012).
[12] X. Y. Li, X. Xiao, H. Xu, Z. Y. Li, T. Chu, J. Z. Yu, Y. D. Yu. Opt. Lett., 38, 1703(2013).
[13] J. J. Xing, Z. Y. Li, Y. D. Yu, J. Z. Yu. Opt. Lett., 38, 4774(2013).
[14] J. J. Xing, Z. Y. Li, P. J. Zhou, X. Xiao, J. Z. Yu, Y. D. Yu. Opt. Lett., 38, 3926(2013).
[15] A. Khilo, C. M. Sorace, F. X. Kärtner. Opt. Express, 19, 4485(2011).