Author Affiliations
1Research Center of Laser Fusion, China Academy of Engineering Physics, Mianyang 621900, China2Collaborative Innovation Center of IFSA (CICIFSA), Shanghai Jiao Tong University, Shanghai 200240, China3Science and Technology on Plasma Physics Laboratory, Mianyang 621900, China4Center for Crystal Research and Development, Key Laboratory of Functional Crystals and Laser Technology, Technical Institute of Physics and Chemistry, Chinese Academy of Sciences, Beijing 100190, China5Graduate School of China Academy of Engineering Physics, Beijing 100088, Chinashow less
Abstract
We report an efficient mid-infrared extracavity optical parametric oscillator (OPO) based on the nonlinear crystal BaGa4Se7 pumped by a diode-side-pumped Q-switched Nd:Y3Al5O12 (Nd:YAG) laser. The maximum pulse energy of 1.03 mJ at 4.25 μm is obtained with the repetition rate of 10 Hz and pulse width of 12.6 ns when the pump energy was 13.5 mJ, corresponding to an optical-to-optical conversion efficiency of 7.6% from 1.064 μm to 4.25 μm. The idler wave slope conversion efficiency was 12%. To the best of our knowledge, it is the highest reported conversion efficiency for the compact BaGa4Se7 OPO driven by the Nd:YAG laser.Recently, the advance in mid-infrared (mid-IR) lasers has gained increasing attentions due to their wide applications in many fields, such as lidar systems, range finders, and remote sensing[1–4]. Solid state lasers are promising candidates for these purposes, allowing for their compactness, high efficiency, and reliability in rugged conditions. Furthermore, many researchers show particular interest in the lasers emitting at the wavelength between and , which coincides with the Earth’s atmosphere optical transmission window[5,6]. For instance, the pulsed laser sources operating in this spectral region are of special importance for many lidar applications. Among the general methods, an optical parametric oscillator (OPO) is an attractive approach, which supplies a simple and efficient means to realize such high-energy lasers[7–12]. In order to down-convert the laser frequency to the mid-IR region, nonlinear optical (NLO) crystals with large NLO coefficients, wide transparent region, and high laser damage threshold are desired for the parametric devices. The recently discovered (BGSe) crystal has been reported as a potential alternative NLO crystal for generating mid-IR radiation[13–19], and has been applied in mid-IR laser systems[20–22]. In addition, the BGSe OPO pumped by Nd-nanosecond laser systems emitting at ∼1 μm has been proved to be an effective way to obtain coherent radiation in the spectral wavelength range of the mid-IR. Kostyukova et al. have reported 3.7 mJ, ∼10 ns, and 10 Hz, ∼7.2 μm mid-IR output in a BGSe nanosecond OPO under 1.064 μm pumping, corresponding to an optical-to-optical conversion efficiency of 5.9% from 1064 nm to the mid-IR output[23]. Recently, a single pump passing singly resonant OPO based on the BGSe crystal pumped by a 1064 nm Q-switched (Nd:YAG) laser has been reported[24]. The maximum pulse energy of the idler wavelength was 2.56 mJ at a 4.11 μm wavelength with 10 Hz repetition rate when the pump energy was 61.6 mJ, corresponding to an optical-to-optical conversion efficiency of 4.16% and with a maximum idler wave slope conversion efficiency of 7.77%.
In this Letter, we report a high-energy and high-efficiency mid-IR output at 4.25 μm from a compact extracavity BGSe OPO pumped by a diode-pumped Q-switched Nd:YAG laser. With the pump energy of 13.5 mJ, pump pulse width of 6.7 ns, and repetition rate of 10 Hz, the output energy of 1.03 mJ and pulse width of 12.6 ns are obtained, corresponding to an optical–optical conversion efficiency of 7.6% from 1.064 μm to 4.25 μm, with the slope conversion efficiency of 12%. To the best of our knowledge, this is the highest reported conversion efficiency in an extracavity BGSe OPO for generating mid-IR radiation driven by the diode-pumped Nd:YAG laser.
BGSe was reported as a promising NLO crystal for practical applications in the mid-IR region[13,14]. The BGSe crystal has the transparent range covering from 0.47 μm to 18 μm, which makes it possible to obtain the mid-IR coherent light through parametric down-conversion. The crystal belongs to the monoclinic system (m-point group). It has a relatively wide bandgap of about 2.64 eV, being beneficial for maintaining high laser induced damage threshold and avoiding the two-photon absorption of a conventional pumping laser at 1064 nm. The Type-I phase matching conditions () for the BGSe crystal were calculated at the x–y plane, where the refractive index characteristics for BGSe were given by Ref. [15]. The phase matching curve of BGSe for the signal and idler pumped at 1064 nm is shown in Fig. 1. It indicated that, within the matching angle range of 58.5°–51.1°, the tuning of the idler from 3 μm to 5 μm corresponded to a signal range of 1649 nm–1352 nm[25]. Specifically, the 4.3 μm idler light was obtained under the Type-I phase matching () condition, which was adopted in our experiment.
Sign up for Chinese Optics Letters TOC. Get the latest issue of Chinese Optics Letters delivered right to you!Sign up now
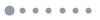
Figure 1.Type-I phase matching curve of the OPO by 1064 nm in BGSe.
The experimental configuration of the BGSe OPO is shown in Fig. 2. The BGSe OPO was pumped by a diode-side-pumped Q-switched pulse Nd:YAG laser with a repetition frequency of 10 Hz. In order to generate the pump light of 1064 nm with good beam quality and short pulse width, we design a plane–plane cavity with the Nd:YAG module side-pumped by an 808 nm diode placed asymmetrically near the former cavity mirror M1 [highly reflective (HR) coated at 1064 nm] and the Pockel cell (PC) near the output cavity mirror M2 [partially reflective (PR) coated at 1064 nm with a transmissivity of 50%]. A quarter-wave plate (QWP) and a polarizer (P) were used to choose the line-polarized light to go through the PC. The cavity length was as short as possible at 200 mm. The driven source of the PC operated at a pulse repetition frequency (PRF) of 10 Hz. The pump pulse was firstly reflected by two 45° plane mirrors, M3 and M4, which were HR coated at 1064 nm (), and subsequently transmitted through a half-wave plate (HWP) and an isolator to avoid the reflected 1064 nm laser beam from the OPO-cavity into the Q-switched module cavity. The HWP and polarized beam splitter (PBS) were employed to get the -polarized light.
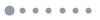
Figure 2.Experimental configuration of the extracavity BGSe OPO.
The BGSe OPO cavity consisted of a flat mirror M5, a BGSe crystal, and a flat output-coupling mirror M6. The cavity length has been optimized to be 50 mm, and the optical length was 103 mm. Input mirror M5 was anti-reflective (AR) coated at 1064 nm on one surface, HR coated at 3.8 μm–4.7 μm, and highly transmissive (HT) coated at 1064 nm on the other surface. The output coupler M6 was HR coated at 1064 nm () to increase the utilization of the pump energy (so as to improve the conversion efficiency), achieving about 20% transmission in the range of 3.8 μm–4.7 μm for the idler to obtain a high output energy. The BGSe crystal had dimensions of , which was cut in the x–z plane at and for o−ee Type-I phase matching, with the 8 mm edge parallel to the axis and polished for both surfaces with AR coatings. Moreover, the crystal was wrapped in indium foil and mounted on a copper block without cooling. In order to separate the idler and signal laser beams, a prism with 30° angle without coating was used near the output-coupling mirror.
The energies of pump and idler light were measured by an energy meter (Ophir PE10-C, metallic probe, with a maximum range of 10 mJ covering the wavelength range from 0.15 μm to 12 μm). The pulse temporal behaviors of the pump and signal light were simultaneously recorded by a Tektronix digital oscilloscope (1 GHz bandwidth, and 5 GSa/s sampling rate) and two fast photo-detectors. Meanwhile, the beam profile of the 1064 nm pump was measured by a high-quality CCD camera (Thorlabs, BC106-VIS).
At first, the performance of the diode-side-pumped Q-switched Nd:YAG laser was studied. The output pulse energy and pulse width of the pump light at 1064 nm are shown in Fig. 3. It can be seen that as the pump current increased from 40 A to 70 A, the output pulse energy would also increase monotonically from 2.6 mJ to 13.5 mJ, while the output pulse width witnessed a decrease from 11 ns to 6.7 ns. At the diode pump current of 70 A, the maximum output pulse energy of 13.5 mJ was obtained, and the pulse width was 6.7 ns. The beam profile of a Gaussian diameter () of 2.6 mm in the position of the OPO at the maximum pump current was obtained, as shown in Fig. 4.
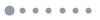
Figure 3.Pulse energy and pulse width at 1064 nm versus the diode pump current of the Q-switched Nd:YAG laser.
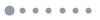
Figure 4.Beam profile of the pump light of 1064 nm.
The spectra of the pump and signal waves were monitored by an optical spectrum analyzer (Ocean Optics, NIRQuest-256, with the wavelength range of 870 nm to 2500 nm). Merely 1% energy could be transmitted through the AR coated output coupler M6, i.e., the residual 1064 nm pump energy was reserved in the output laser beam of the OPO cavity. Both the pump wave and signal wave are measured simultaneously at the same position behind mirror M6. As shown in Fig. 5, the typical spectral peaks of the pump and signal waves are at 1064 nm and 1419 nm, respectively. According to the OPO momentum conservation condition (), the measured pump wavelength is 1064 nm, the signal wavelength is 1419 nm, and, in this case, the idler wavelength is calculated to be 4.25 μm.
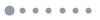
Figure 5.Spectrum of the pump and signal waves.
The energy of the 4.25 μm idler output versus the 1064 nm pump energy is shown in Fig. 6. As depicted in the curve, the output idler energy increases with the increasing injected pump energy. The threshold pulse energy was about 5 mJ, which corresponds to the energy fluence of and the peak intensity of . For reference, it is higher than that in Ref. [24], owing to the longer cavity length and no HR coated output mirror at the signal wave. However, the higher threshold pump energy should lead to a higher saturation pump energy, which would bring higher conversion efficiency in the OPO. The maximum output energy of 1.03 mJ at 4.25 μm was obtained under the pump energy of 13.5 mJ, corresponding to an optical–optical conversion efficiency of 7.6% from 1.064 μm to 4.25 μm, which was the highest conversion efficiency in a BGSe OPO for the 4.25 μm mid-IR light, to the best of our knowledge. In addition, the pump pulse duration and beam diameter were 6.7 ns and 2.6 mm, respectively. The corresponding maximum power intensity was estimated to be up to , which was higher than the former work[24] and still lower than the damage threshold of the BGSe crystal. The idler wave slope conversion efficiency was about 12%, which was calculated from the linear fitting curves. As seen, the saturation effect, which was caused by the back conversion, had not happened. The energy of the pump could not improve further, owing to the output ability of the existing homemade Q-switched pulse Nd:YAG laser.
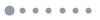
Figure 6.Output pulse energy at 4.25 μm idler light versus the pump (1064 nm) pulse energy.
Under the OPO energy conservation condition (), the signal and the idler photons are generated in pairs. Debuisschert et al.[26] presented that the idler output performance is entirely driven by the signal and pump fields through the nonlinear medium. Miao et al.[27] assumed that the pulses of the two parametric types of radiation (signal and idler waves) have the same temporal property. Owing to the lack of an appropriate detector, we monitor the signal wave from the OPO cavity to indicate the temporal behavior of the idler wave. Figure 7 illustrates the temporal profiles of the pump and signal pulses under the pump energy of 13.5 mJ. The pump pulse duration is monitored by a fast photo-detector (rise time , 170 nm–1100 nm), placed near the P in the Q-switched Nd:YAG cavity to monitor the reflected 1064 nm laser light. The signal pulse duration is monitored by the other fast photo-detector (rise time , 350 nm–1700 nm). The detector is placed behind the prism, and employed a 45° HR mirror ( at 1064 nm) to avoid the residual 1064 nm light. Both collected temporal signals are recorded by a Tektronix digital oscilloscope. The measured pump pulse duration is 6.7 ns, and the signal pulse duration is 12.6 ns, which is equal to the duration of idler light. As shown in Fig. 7, the interesting pulse lengthening phenomenon was observed in our experiments, and the output idler pulse duration is about two times longer than the injected pump pulse width. The in-depth theoretical studies for it will be carried out in our future work.
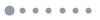
Figure 7.Measured temporal pulse shapes of the 1064 nm pump wave and 1.419 μm signal wave for BGSe-II at a pump energy of 13.5 mJ.
In summary, we have demonstrated high-energy and high-efficiency mid-IR output at 4.25 μm from an extracavity BaGSe OPO pumped by a Q-switched Nd:YAG laser at 1064 nm. The maximum output pulse energy of 1.03 mJ at 4.25 μm was obtained from the BGSe OPO with a repetition rate of 10 Hz. The optical–optical conversion efficiency from 1.064 μm to 4.25 μm was 7.6%, and the slope conversion efficiency was 12%, which is the highest reported conversion efficiency in a BGSe OPO for the 4.25 μm light, to the best of our knowledge. Furthermore, the pulse lengthening phenomenon was observed in our experiment, i.e., the output pulse duration is 12.6 ns with 13.5 mJ pumping energy, being about two times that of the injected pump pulse width.
References
[1] E. Lippert, S. Nicolas, G. Arisholm, K. Stenersen, G. Rustad. Appl. Opt., 45, 3839(2006).
[2] A. Schliesser, N. Picqué, T. W. Hänsch. Nat. Photon., 6, 440(2012).
[3] M. E. Doroshenko, H. Jelınkova, P. Koranda, J. Sulc, T. T. Basiev, V. V. Osiko, V. K. Komar, A. S. Gerasimenko, V. M. Puzikov, V. V. Badikov, D. V. Badikov. Laser Phys. Lett., 7, 38(2010).
[4] Z. P. Qin, G. Q. Xie, J. G. Ma, P. Yuan, L. J. Qian. Chin. Opt. Lett., 15, 111402(2017).
[5] T. T. Basiev, Yu. V. Orlovskii, B. I. Galagan, M. E. Doroshenko, I. N. Vorob’ev, L. N. Dmitruk, A. G. Papashvili, V. N. Skvortsov, V. A. Konyushkin, K. K. Pukhov, G. A. Ermakov, V. V. Osiko, A. M. Prokhorov, S. Smith. Laser Phys., 12, 859(2002).
[6] S. D. Velikanov, N. A. Zaretskiy, E. A. Zotov, V. I. Kozlovsky, Yu. V. Korostelin, O. N. Krokhin, A. A. Maneshkin, Yu. P. Podmar’kov, S. A. Savinova, Ya. K. Skasyrsky, M. P. Frolov, R. S. Chuvatkin, I. M. Yutkin. Quantum Electron., 45, 1(2015).
[7] X. L. Dong, B. T. Zhang, J. L. He, H. T. Huang, K. J. Yang, J. L Xu, C. H. Zuo, S. Zhao, G. Qiu, Z. K. Liu. Opt. Commun., 282, 1668(2009).
[8] V. Petrov, P. G. Schunemann, K. T. Zawilski, T. M. Pollak. Opt. Lett., 34, 2399(2009).
[9] A. Tyazhev, V. Vedenyapin, G. Marchev, L. Isaenko, D. Kolker, S. Lobanov, V. Petrov, A. Yelisseyev, M. Starikova, J. J. Zondy. Opt. Mater., 35, 1612(2013).
[10] Y. Y. Li, K. Yang, G. Y. Liu, B. Q. Yao, Y. L. Ju. Chin. Opt. Lett., 17, 081404(2019).
[11] E. C. Ji, M. M. Nie, Q. Liu. Chin. Opt. Lett., 15, 091402(2017).
[12] J. H. Yuan, C. Li, B. Q. Yao, J. Y. Yao, X. M. Duan, Y. Y. Li, Y. J. Shen, Y. C. Wu, Z. Cui, T. Y. Dai. Opt. Express, 24, 6(2016).
[13] J. Y. Yao, D. J. Mei, L. Bai, Z. S. Lin, W. L. Yin, P. Z. Fu, Y. C. Wu. Inorg. Chem., 49, 9212(2010).
[14] J. Y. Yao, W. L. Yin, K. Feng, X. X. Li, D. J. Mei, Q. M. Lu, Y. B. Nie, Z. W. Zhang, Z. G. Hu, Y. C. Wu. J. Cryst. Growth., 346, 1(2012).
[15] F. Yang, J. Y. Yao, H. Y. Xu, F. F. Zhang, N. X. Zhai, Z. H. Lin, N. Zong, Q. J. Peng, J. Y. Zhang, D. F. Cui, Y. C. Wu, C. T. Chen, Z. Y. Xu. IEEE Photon. Technol. Lett., 27, 1100(2015).
[16] V. Badikov, D. Badikov, G. Shevyrdyaeva, A. Tyazhev, G. Marchev, V. Panyutin, V. Petrov, A. Kwasniewski. Phys. Status Solidi RRL., 5, 31(2011).
[17] E. Boursier, P. Segonds, J. Debray, P. L. Inácio, V. Panyutin, V. Badikov, D. Badikov, V. Petrov, B. Boulanger. Opt. Lett., 40, 4591(2015).
[18] X. Zhang, J. Y. Yao, W. L. Yin, Y. Zhu, Y. C. Wu, C. T. Chen. Opt. Express, 23, 552(2015).
[19] Y. W. Guo, Z. Li, Z. T. Lei, X. Y. Luo, J. Y. Yao, C. H. Yang, Y. C. Wu. Cryst. Growth Des., 19, 1282(2019).
[20] B. Zhao, Y. Chen, B. Yao, J. Yao, Y. Guo, R. Wang, T. Dai, X. Duan. Opt. Mater. Express, 8, 3332(2018).
[21] S. W. Hu, L. Wang, Y. W. Guo, T. Q. Cheng, X. Y. Wu, Z. Y. Wang, H. X. Wu, J. Y. Yao, Y. C. Wu, H. H. Jiang. Opt. Lett., 44, 2201(2019).
[22] M. G. Sun, Z. S. Cao, J. Y. Yao, H. L. Ma, Y. W. Guo, X. M. Gao, R. Z. Rao, Y. C. Wu. Opt. Express, 27, 4014(2019).
[23] N. Y. Kostyukova, A. A. Boyko, V. Badikov, D. Badikov, G. Shevyrayaeva, V. Panyutin, G. M. Marchev, D. B. Kolker, V. Petrov. Opt. Lett., 41, 3667(2016).
[24] W. T. Xu. Appl. Phys. B, 123, 80(2017).
[25] . Opt. Lett., 38, 3903(2013).
[26] . J. Opt. Soc. Am. B, 13, 1569(1996).
[27] . Appl. Opt., 47, 4287(2008).