Abstract
The goal of this work is to evaluate the compositional and morphological changes of human dentin after erbium, chromium:yttrium–scandium–gallium–garnet (Er,Cr:YSGG) laser irradiation by Raman spectroscopy. The dentin surface of human molars are irradiated with an Er,Cr:YSGG laser at the output power of 3 and 3.5 W. Raman spectra before and after treatments are measured and analyzed. The results show that Raman spectroscopy can efficiently monitor the compositional changes of human dentin induced by an Er,Cr:YSGG laser. Although no new bands, band shifts, or disappearance of bands occurred, the intensities of the organic peaks associated with Amide I and are reduced significantly.Since the invention of the first laser by Maiman in 1960, there has been great interest among various researchers to use this tool to make dental treatment more comfortable. Ruby, argon, , and neodymium:yttrium aluminum garnet (Nd:YAG) had been investigated in early studies and showed the potential to have a real effect in clinical dentistry. However, at the same time, major thermal effects came with the use of lasers, including melting, carbonization, the creation of fissures and cracks in the surrounding tissue, as well as an increase in pulp temperature[1,2]. With the discovery of erbium (Er) lasers, especially the erbium, chromium:yttrium–scandium–gallium–garnet (Er,Cr:YSGG) laser, they can realize nearly pain-free cutting without the need for a local anesthetic and without causing critical damage, and thus are considered the most promising alternatives to traditional mechanical instruments for the preparation of various tooth structures[3,4]. The Er,Cr:YSGG laser with a wavelength of 2.78 μm, which is exactly within the major absorption band of water, produces a room temperature absorption coefficient () of . The absorbed energy is thought to cause rapid vaporization of water and other hydrated organic components of the tissues and produces micro-explosions. Due to vaporization, the internal pressure builds in the tissue until it reaches the ultimate tensile strength of the inorganic substance before the melting point is achieved, consequently resulting in the removal of dental hard tissue.
The changes of chemical content and microstructure of human dentine play an important role in the prevention and therapy of dental disease. According to different dental treatments, corresponding laser parameters and irradiation conditions must be selected, thus inducing appropriate chemical and morphological effects. For example, when laser treatment for hypersensitive dentine is desired, the dentine tubules must be fused and sealed, such that the stimulation from the outside can be cut off. For caries removal and restoration, the dentin tubules must be opened, rather than closed, in favor of adhesion between the tooth and the restorative materials. For caries prevention, the laser light must alter the composition or solubility of the dental substrate and increase its acid resistance. Knowledge of the changes of chemical content and microstructure of human dentine constitutes the basis for laser use in dentistry.
Raman spectroscopy is a promising vibrational spectroscopic technique which provides information about the biochemical composition and molecular structure, also called the “molecular fingerprint.” Since it can easily obtain highly specific biochemical information about protein, lipids, and mineral orientation and composition, Raman spectroscopy is well-suited to access mineralized tissues, such as bone and dental hard tissues. Camerlingo et al.[5] showed that compared with conventional mechanical methodologies, laser treatment affected the spectrum of organic components of the dentine. Moreover, these changes depended on the time duration of the laser pulse used. Not only in the organic component, greater reduction of inorganic contents in the areas of Raman peak was also observed after erbium-doped:YAG (Er:YAG) laser irradiation on dentin[6]. In contrast, the use of Er:YAG laser at 12 J did not affect the dentin components in samples treated with thymol[7]. It should be pointed out that the type of decontamination method used for teeth studied in vitro may alter the chemical characteristics of teeth. Due to diverse irradiation parameters and characteristics of biological tissues, there are some controversies on the effect of laser irradiation on hard dental tissue. Compared to the Er:YAG laser, less is known about the compositional changes induced by the Er,Cr:YSGG laser and this topic has been getting more and more attention[8].
Sign up for Chinese Optics Letters TOC. Get the latest issue of Chinese Optics Letters delivered right to you!Sign up now
This work evaluates the changes of chemical content and microstructure of human dentine after Er,Cr:YSGG laser irradiation by Raman spectroscopy and a scanning electron microscope (SEM).
Noncarious human molars with completed root growth, which were removed by extraction or osteotomy for medical reasons, were collected from healthy subjects (18–30 years old). After extraction, the remaining soft tissue was removed from the tooth surface and the teeth were thoroughly washed in tap water. Vertical sectioning was done by using a diamond wheel saw (Model 650, South Bay Technology Inc., United States) under running water. Each tooth was cut into two or three slices ( thickness), where the slices at both sides of the tooth were abandoned and that near the center of the tooth remained. The exposed surface of the slabs was grounded by a water-cooled polishing machine using a series of silicon carbide sandpapers of 240–1200 grit followed by ultrasonic cleaning. All the prepared slices were stored in physiological saline at 4°C until use.
An Er,Cr:YSGG laser (Waterlase, Biolase Technology, United States) was coupled with an optic fiber system and transmitted into a handpiece consisting of a Z6 sapphire tip with a diameter of 600 μm. Laser energy before and after each experiment was calibrated by an energy meter (NOVA II, Orphir, Israel) equipped to a pyroelectric detector. An Er,Cr:YSGG laser was applied at the output power of 3 and 3.5 W with a pulse repetition rate of 20 Hz and pulse width of 140 μs. When the measured energy had decreased by over 15% of the initial energy during our work, the tip was replaced. In addition, a built-in pressurized water spray system with an adjustable flow rate was incorporated into the handpiece. Based on pre-experimental results, the air pressure and water level were set at 60% and 70%, respectively. The sample was put on a personal computer (PC)-controlled motorized linear drive stage and the work distance was approximately 1 mm. The schematic description of the experimental setup is shown in Fig. 1. The irradiated area focused on the middle dentine.
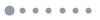
Figure 1.Schematic description of the experimental setup.
Raman spectra were recorded using a Renishaw InVia micro-Raman system with a objective. A multi-element 785 nm diode laser (about 20 mW of power) was used for excitation and Raman spectra were acquired with the integration time of 20 s from 300 to . Raman spectra were measured before and after each treatment. Measurements were taken randomly at three points and the location was kept unaltered during two measurements. All the Raman spectra were extracted out using the program previously described by Zeng et al.[9], and then each measured spectra were normalized to the integrated area. All experimental data were input into a computer and the software SPSS13.0 was used in the statistical analysis. The mean changes in peak intensity before and after treatments were analyzed by the Student–Newman–Keuls (SNK) test following one-way analysis of variance (ANOVA). A 5% level of statistical significance was set. Detailed structure changes of the irradiated areas were further examined by a SEM (JSM-6380LV, Japan).
Figure 2 shows typical Raman spectra of normal dentin. It contains two characteristic parts: (a) vibration modes of phosphate spanning from 400 to ; (b) organic grouping vibration modes in the 1200 to region. Peaks due to inorganic mineral component were obviously exhibited at wavenumber shifts of 430, 581, 960, and . The strongest peak at arose from and its full–width half–maximum (FWHM) was approximately , which was consistent with previous results[10,11]. The peaks at 430 and were assigned to and , respectively. The peak at was attributed to Type B modes of carbonate vibrations. In the Raman spectra of organic components, the bands at 1668 and were assigned to Amide I and vibrations, respectively.
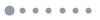
Figure 2.Raman spectra of normal dentin.
After comparison of Raman spectra before and after Er,Cr:YSGG laser treatment, although no new bands, bands shifts, or disappearance of bands occurred in the irradiation process, the peak intensity changed (Figs. 3 and 4). As shown in Fig. 3, the peak intensity at increased and declined slightly at 960 and . The statistical analysis demonstrated that the aforementioned difference was not statistically significant . So far, laser application for dental treatments can be roughly divided into three groups: caries preventive therapy, caries removal accompanied with cavity preparation, and endodontic surgery. For caries inhibition, carbonate loss from the carbonated hydroxyapatite mineral must be presented and converted into a low-solubility hydroxyapatite-like calcium phosphate, thus improving the acid resistance. In addition, dental hard tissue application depended largely on laser parameters, such as wavelength, power, and pulse duration. The dosimetry used in the work was suited to prepare dental cavities. The thermo-mechanical ablation mechanism determined that laser irradiation did not necessarily alter dentin inorganic content. During the ablation process, water and relative components were merely vaporized, and thermal analysis demonstrated the temperature rise did not reach the melting point of the inorganic substance[12,13]. However, the measurement by inductively coupled plasma–atomic emission spectrometry (ICP–AES) indicated that mean percentage weights of calcium (Ca), magnesium, sodium, phosphorus (P), and the Ca/P ratios were affected by laser irradiation[14].
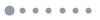
Figure 3.Dentin Raman spectra of inorganic components after Er,Cr:YSGG laser irradiation.
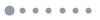
Figure 4.Dentin Raman spectra of organic components after Er,Cr:YSGG laser irradiation.
In Fig. 4 we can observe the intensities of the peaks associated with Amide I and reducing dramatically after laser irradiation. Furthermore, the laser effect on the organic content was found to be statistically significant . The intensity reduction of organic band indicated the decrease of organic matter and suggested that laser treatment may cause thermal decomposition of the carbonated apatite and the organic matter. In thermal analysis, water is the first chemical compound released from the tissue on heating. It could be totally released at 200°C and the organic material released in the temperature range of 100°C–400°C[15]. Although being a minor component, organic matrix may have a great potential in controlling the diffusion and dissolution pathway, and thus prevent demineralization and caries. Another compound released from the tissue is the carbonate radical. The loss in dentine begins at 100°C, more intensive at 700°C, and totally released around 1000°C.
Once a certain laser energy (ablation threshold) is achieved, dentin can be ablated by Er,Cr:YSGG laser. As expected, ablation occurred more markedly at the center of the beam compared with the edge of the crater at a microscopic level and resulted in a corrugated profile. The gradient of the crater side grew up with increasing laser power. As shown in Fig. 5, open dentinal tubules and less debris can be observed after Er,Cr:YSGG irradiation, which helped the adhesive systems to infiltrate the inner part of the dental hard tissue and improve the cohesive force between the tooth and adhesion agent. In addition, the peritubular dentin protruded slightly from the surrounding intertubular dentin. This pattern was thought to be induced by the difference of their content, where the intertubular dentin contained more water and less mineral content than the peritubular, thus causing the intertubular dentin to be selectively ablated to a greater degree.
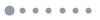
Figure 5.SEM images of dentin after irradiation by a pulsed Er,Cr:YSGG laser: (a) 3 and (b) 3.5 W.
In conclusion, Raman spectroscopy analysis show that the Er,Cr:YSGG laser produces a strong modification of the dentin organic components and these changes are identified by the decrease of the Amide I peak at and vibrations at . SEM images suggest that the morphology after laser irradiation may be beneficial to the subsequent adhesion of dental restorative materials.
References
[1] H. A. Wigdor, J. J. Walsh, J. D. Featherstone, S. R. Visuri, D. Fried, J. L. Waldvogel. Lasers Surg. Med., 16, 103(1995).
[2] J. D. B. Featherstone, D. Fried. Med Laser Appl., 16, 181(2001).
[3] S. Parker. Brit. Dent. J., 202, 445(2007).
[4] P. Ekworapoj, S. K. Sidhu, J. F. McCabe. Lasers Med. Sci., 22, 175(2007).
[5] C. Camerlingo, M. Lepore, G. M. Gaeta, R. Riccio, C. Riccio, A. De Rosa, M. De Rosa. J. Dent., 32, 399(2004).
[6] L. E. Soares, J. A. Brugnera, F. A. Zanin, M. T. Pacheco, A. A. Martin. Photomed. Laser Surg., 25, 50(2007).
[7] L. E. Soares, E. S. A. Do, A. B. Junior, F. A. Zanin, S. C. C. Da, R. de Oliveira, A. A. Martin. J. Biomed. Opt., 14, 24001(2009).
[8] P. A. Ana, C. M. F. Kauffmann, L. Bachmann, L. E. S. Soares, A. A. Martin, A. S. L. Gomes, D. M. Zezell. Laser Phys., 24, 035603(2014).
[9] J. Zhao, H. Lui, D. I. McLean, H. Zeng. Appl. Spectrosc., 61, 1225(2007).
[10] P. Tramini, B. Pelissier, J. Valcarcel, B. Bonnet, L. Maury. Caries Res., 34, 233(2000).
[11] A. C. Ko, L. P. Choo-Smith, M. Hewko, L. Leonardi, M. G. Sowa, C. C. Dong, P. Williams, B. Cleghorn. J. Biomed. Opt., 10, 31118(2005).
[12] J. A. Izatt, D. Albagli, M. Britton, J. M. Jubas, I. Itzkan, M. S. Feld. Lasers Surg. Med., 11, 238(1991).
[13] M. Lin, F. Xu, T. J. Lu, B. F. Bai. Dent. Mater., 26, 501(2010).
[14] A. Secilmis, S. Altintas, A. Usumez, G. Berk. Lasers Med. Sci., 23, 421(2008).
[15] L. Bachmann, R. Diebolder, R. Hibst, D. M. Zezell. Spectrochim. Acta A, 61, 2634(2005).