
- Chinese Optics Letters
- Vol. 20, Issue 10, 100602 (2022)
Abstract
1. Introduction
With the rapid development of Internet, cloud computing, Internet of Things, and other technologies, the conventional erbium-doped fiber amplifiers (EDFAs), which work mainly in the C + L band, cannot meet the growing demand from the capacity of optical fiber communication systems. Thus, it is urgent to research and develop optical fiber amplifiers outside the C + L band. At present, some rare earth-doped fiber amplifiers have been developed, such as praseodymium-doped[
Studies have shown that luminescence properties of Bi-doped silica fibers are closely related to the core composition and the valence state of Bi ions[
In this paper, we developed a fiber amplifier operating at 1355–1385 nm using a home-made low-loss Bi/P co-doped silica fiber (BPDF). The amplifying performance at different pumping conditions and fiber lengths was compared, and the gain and noise figure (NF) characteristics with different pump and signal powers were evaluated. Using a bidirectional pumping configuration with two 1240 nm LDs, the maximum gain about 20 dB and the minimum NF of 4.6 dB at 1355 nm were achieved with a total pump power of 870 mW and signal power of
Sign up for Chinese Optics Letters TOC. Get the latest issue of Chinese Optics Letters delivered right to you!Sign up now
2. Experiment and Methods
The BPDF was fabricated by the modified chemical vapor deposition (MCVD) technique combined with the solution doping method. The core and cladding diameters were 6 and 125 µm, respectively. The numerical aperture and cutoff wavelength were 0.14 and
The experimental setup of the single stage bidirectional pumping BPDF is schematically shown in Fig. 1. A tunable laser source (TLS, Santec) operating in the range of 1355–1485 nm was used as the input signal source with an isolator (ISO) and attenuator (ATT). Two LDs at 1240 and 1280 nm, each with up to
Figure 1.Experimental setup of the single stage BPDF amplifier. Points 1 and 2 are fusion splicing joints between BPDF and WDMs.
3. Result and Discussion
Figure 2(a) shows the background loss of the BPDF. The background loss at 1550 nm was as low as
Figure 2.(a) Background loss and (b) absorption spectrum of the BPDF.
As shown in Fig. 3, two amplified spontaneous emission (ASE) spectra of 190 m BPDF were measured with 1240 and 1280 nm LDs (415 mW pump power). Under the excitation of 1240 or 1280 nm, the ASE spectra both showed a broad band with different peak positions. The peak position of the ASE spectrum under 1240 nm excitation was at
Figure 3.ASE spectra of the BPDF at 1240 and 1280 nm pumping.
Then, the gain performances of the BPDF pumped by a 1240 nm LD with bidirectional, forward, and backward pumping configurations were compared. The total pump and signal powers of each pumping configuration were 415 mW and
Figure 4.Gain characteristics of three pumping configurations, bidirectional pumping with the fiber length of 190 m and forward and backward pumping with the fiber length of 170 m.
Figure 5 shows the gain performances of three different kinds of pumping wavelength combinations with the bidirectional pumping configuration. The total pump power for each combination was fixed to be 415 mW, and the pump power for forward and backward was equal to a 3 dB optical fiber coupler. For a given total pump power, the gain from 1355 to 1370 nm was the highest with two 1240 nm LDs pumping, while that from 1370 to 1385 nm was the highest with two 1280 nm LDs pumping. Therefore, the result illustrated that using two 1280 nm LDs benefited the achievement of gain at a longer wavelength, whilst improving gain flatness. Using two 1240 nm LDs was beneficial for improving the gain at a shorter wavelength, and a maximum gain of
Figure 5.Gain characteristics under different pump wavelength combinations at fixed pump and signal power as well as fiber length.
The optical gain and NF characteristics of BPDF were measured by bidirectional pumping with two 1240 nm LDs, as shown in Fig. 6. It was clear that the optimal length of the fiber was 190 m for getting the maximum gain at 1355 nm [see Fig. 6(a)]. However, limited by the signal source operating at shorter wavelength and the matched WDMs, we cannot further optimize the maximum gain value at shorter wavelength. The gain and NF at different input signal powers of 1355 nm with the fixed pump power of 870 mW are shown in Fig. 6(b). The input signal was recorded before the BPDF (point 1, see Fig. 1), and thus the NF was calculated without considering the insertion loss of the first WDM (0.5 dB). There was no obvious difference of the gain and NF with different input signal powers of
Figure 6.Gain and NF characteristics under bidirectional pumping with two 1240 nm LDs. (a) Gain versus fiber length with the pump power of 415 mW and the input signal power of −30 dBm. (b) Gain or NF versus signal power with the fiber length of 190 m and the pump power of 870 mW. (c) Gain or NF versus pump power with the fiber length of 190 m and the input signal power of −30 dBm.
4. Conclusion
In this paper, we prepared a low-loss BPDF by MCVD technology, where the background loss at 1550 nm was as low as 17 dB/km. We demonstrated for the first time, to the best of our knowledge, an all-fiber amplifier successfully operating in the E-band using this home-made BPDF. We systemically compared the gain performance of different pumping methods. The bidirectional pumping was of great benefit to achieving higher gain than either forward or backward pumping. The gain characteristics with different pump or signal powers were evaluated. A maximum gain at 1355 nm close to 20 dB and a minimum NF of 4.6 dB were obtained using two 1240 nm LDs under bidirectional pumping with the input pump and signal powers of 870 mW and
References
[1] Y. Miyajima, T. Sugawa, Y. Fukasaku. 38.2 dB amplification at 1.31 µm and possibility of 0.98 µm pumping in Pr3+-doped fluoride fibre. Electron. Lett., 27, 1706(1991).
[2] M. Brierley, S. Carter, P. France, J. E. Pedersen. Amplification in the 1300 nm telecommunications window in a Nd-doped fluoride fiber. Electron. Lett., 26, 329(1990).
[3] S. Chen, Y. Jung, S. U. Alam, R. Sidharthan, D. Ho, S. Yoo, D. J. Richardson, J. M. O. Daniel. Ultra-short wavelength operation of thulium-doped fibre amplifier in the 1628–1655 nm waveband. European Conference on Optical Communication(2018).
[4] J. Bromage. Raman amplification for fiber communications systems. J. Light. Technol., 22, 79(2004).
[5] Y. Fujimoto, M. Nakatsuka. Infrared luminescence from bismuth-doped silica glass. Jpn. J. Appl. Phys., 40, L279(2001).
[6] S. V. Firstov, V. F. Khopin, I. A. Bufetov, E. G. Firstova, A. N. Guryanov, E. M. Dianov. Combined excitation-emission spectroscopy of bismuth active centers in optical fibers. Opt. Express, 19, 19551(2011).
[7] I. A. Bufetov, M. A. Melkumov, S. V. Firstov, K. E. Riumkin, A. V. Shubin, V. F. Khopin, A. N. Guryanov, E. M. Dianov. Bi-doped optical fibers and fiber lasers. IEEE J. Sel. Top. Quantum Electron., 20, 111(2014).
[8] N. K. Thipparapu, Y. Wang, S. Wang, A. A. Umnikov, P. Barua, J. K. Sahu. Bi-doped fiber amplifiers and lasers. Opt. Mater. Express, 9, 2446(2019).
[9] A. Donodin, V. Dvoyrin, E. Manuylovich, L. Krzczanowicz, W. Forysiak, M. Melkumov, V. Mashinsky, S. Turitsyn. Bismuth doped fibre amplifier operating in E- and S- optical bands. Opt. Mater. Express, 11, 127(2021).
[10] Y. Ososkov, A. Khegai, S. Firstov, K. Riumkin, S. Alyshev, A. Kharakhordin, A. Lobanov, A. Guryanov, M. Melkumov. Pump-efficient flattop O+E-bands bismuth-doped fiber amplifier with 116 nm -3 dB gain bandwidth. Opt. Express, 29, 44138(2021).
[11] Y. Wang, N. K. Thipparapu, D. J. Richardson, J. K. Sahu. Ultra-broadband bismuth-doped fiber amplifier covering a 115-nm bandwidth in the O and E bands. J. Light. Technol., 39, 795(2021).
[12] N. K. Thipparapu, S. Jain, A. A. Umnikov, P. Barua, J. K. Sahu. 1120 nm diode-pumped Bi-doped fiber amplifier. Opt. Lett., 40, 2441(2015).
[13] Y. Wang, N. K. Thipparapu, S. Wang, P. Barua, D. J. Richardson, J. K. Sahu. Study on the temperature dependent characteristics of O-band bismuth-doped fiber amplifier. Opt. Lett., 44, 5650(2019).
[14] V. V. Dvoyrin, V. M. Mashinsky, S. K. Turitsyn. Bismuth-doped fiber amplifier operating in the spectrally adjacent to EDFA range of 1425–1500 nm. Optical Fiber Communication Conference(2020).
[15] E. M. Dianov, S. V. Firstov, S. V. Alyshev, A. V. Kharakhordin, K. E. Riumkin, M. A. Melkumov, V. F. Khopin, A. N. Guryanov. Bismuth-doped optical fibers for the telecommunication L and U bands: effect of laser and thermal treatment on gain characteristics. European Conference on Optical Communication (ECOC)(2018).
[16] M. Y. Peng, J. R. Qiu, D. P. Chen, X. G. Meng, I. Y. Yang, X. W. Jiang, C. S. Zhu. Bismuth- and aluminum-codoped germanium oxide glasses for super-broadband optical amplification. Opt. Lett., 29, 1998(2004).
[17] S. F. Zhou, H. F. Dong, H. P. Zeng, G. F. Feng, H. C. Yang, B. Zhu, J. R. Qiu. Broadband optical amplification in Bi-doped germanium silicate glass. Appl. Phys. Lett., 91, 061919(2007).
[18] C. Jiang. Modeling a broadband bismuth-doped fiber amplifier. IEEE J. Sel. Top. Quantum Electron., 15, 79(2009).
[19] Y. Luo, J. Wen, J. Zhang, J. Canning, G. D. Peng. Bismuth and erbium codoped optical fiber with ultrabroadband luminescence across O-, E-, S-, C-, and L-bands. Opt. Lett., 37, 3447(2012).
[20] S. Zhou, H. Dong, H. Zeng, J. Hao, J. Chen, J. Qiu. Infrared luminescence and amplification properties of Bi-doped GeO2−Ga2O3−Al2O3 glasses. J. Appl. Phys., 103, 103532(2008).
[21] J. Wu, D. Chen, X. Wu, J. Qiu. Ultra-broad near-infrared emission of Bi-doped SiO2-Al2O3-GeO2 optical fibers. Chin. Opt. Lett., 9, 071601(2011).
[22] Y.-S. Wang, Z.-W. Jiang, H.-X. Luan, Z.-X. Zhang, J.-G. Peng, L.-Y. Yang, J.-Y. Li, N.-L. Dai. Preparation and spectral characteristics of Bi-doped double cladding fiber. Acta Phys. Sin., 61, 084215(2012).
[23] Y.-S. Wang, Z.-W. Jiang, J.-G. Peng, H.-Q. Li, L.-Y. Yang, J.-Y. Li, N.-L. Dai. Controlling the spectral characteristics of bismuth doped silicate glass based on the reducing reaction of Al powder. Chin. Phys. Lett., 30, 044207(2013).
[24] J. Wu, X. Wu. Fabrication of Er/Bi Co-doped silica preforms with a broadband infrared emission. Opto-Electron, 4, 6(2014).
[25] P. Liu, L. Liao, Y.-B. Chu, Y.-B. Wang, X.-W. Hu, J.-G. Peng, J.-Y. Li, N.-L. Dai. Irradiation and temperature influence on the Bi-doped silica fiber. Acta Phys. Sin., 64, 224220(2015).
[26] J. Cao, Z. Zhang, X. Li, M. Peng. Abnormal NIR photoemission from bismuth doped germanophosphate photonic glasses. J. Mater. Chem. C, 7, 3218(2019).
[27] Q. Zhao, Q. Hao, Y. Luo, G. D. Peng. Thermal-induced luminescence enhancement of BAC-P in bismuth-doped phosphogermanosilicate fibers. Opt. Lett., 45, 1152(2020).
[28] A. Khegai, Y. Ososkov, S. Firstov, K. Riumkin, S. Alyshev, A. Kharakhordin, E. Firstova, F. Afanasiev, V. Khopin, A. Guryanov, M. Melkumov. O-Band bismuth-doped fiber amplifier with 67 nm bandwidth. Optical Fiber Communications Conference and Exhibition (OFC), 3(2020).
[29] W. Yu, N. Kumar Thipparapu, D. J. Richardson, J. Sahu. Bi-doped fiber amplifiers for ultra-wideband optical communication systems. IEEE Photonics Society Summer Topicals Meeting Series (SUM), 3(2021).
[30] P. C. Becker, N. A. Olsson, J. R. Simpson. Erbium-Doped Fiber Amplifiers: Fundamentals and Technology(1999).
[31] E. M. Dianov. Bismuth-doped optical fibers: a challenging active medium for near-IR lasers and optical amplifiers. Light Sci. Appl., 1, e12(2012).
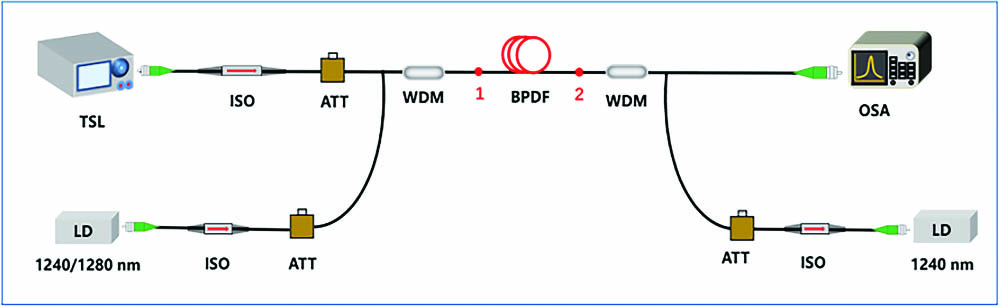
Set citation alerts for the article
Please enter your email address