
- Chinese Optics Letters
- Vol. 21, Issue 3, 030101 (2023)
Abstract
Keywords
1. Introduction
Aerosol is an important component of air pollutants and one of the most uncertain factors in environmental and climate change, and it is closely related to human activities[1–4]. Accurate measurements of aerosol optical properties can help human understand the dynamic change of atmosphere and provide the theoretical reference for weather forecasting and the analysis of global climate change[5,6].
High-spectral-resolution lidar (HSRL) has proven to be a powerful tool for the measurement of aerosol optical properties[7,8], which uses the seeded high-power pulsed laser as the excitation light source, and chooses the narrowband filters (atomic/molecular absorption filter or interferometer optical filter) as the spectral discriminator to separate aerosol Mie scattering signals and molecular Rayleigh scattering signals excited by single-frequency laser pulses[9,10]. The concept of the multilongitudinal mode (MLM) HSRL has been proposed recently[11–14], which abandons the employment of the complex seeded laser techniques, and directly utilizes the high-power pulsed laser with the MLM laser as the excitation light source. Different from the single-frequency HSRL, the MLM-HSRL can only use the optical interferometer with the periodic transmittance function as the spectral discriminator to separate aerosol Mie scattering signals and molecular Rayleigh scattering signals excited by the MLM laser pulses.
Xi’an University of Technology has been developing an MLM-HSRL for aerosol measurements that uses a tunable Mach–Zehnder interferometer (MZI) with a large optical path difference (OPD) as the spectral discriminator[15]. However, the optical match between the free spectral range (FSR) of MZI and the longitudinal mode interval of the MLM laser is frequently influenced by external environmental factors such as laboratory temperature, pressure, and vibration, which leads to a worse discrimination effect of aerosol Mie scattering signals and molecular Rayleigh scattering signals. In order to enhance the discrimination capability of the MZI with large OPD in the MLM-HSRL system, we develop an MZI with self-tuning functionality of the OPD that achieves the dynamic match in nanometer magnitude between the FSR of MZI and the longitudinal mode interval of the MLM lasers by using the piezoelectric ceramic nanodisplacement platform. In this paper, we present the techniques of the MLM-HSRL with self-tuning MZI and its preliminary measurements of aerosol optical properties.
Sign up for Chinese Optics Letters TOC. Get the latest issue of Chinese Optics Letters delivered right to you!Sign up now
2. Setup of the MLM-HSRL and Data Retrieval
The MLM-HSRL system based on the self-tuning MZI is shown in Fig. 1. The Nd:YAG high-power pulsed laser with a wavelength of 532 nm and a pulse energy of 150 mJ at the repetition rate of 10 Hz is used as the transmitter. The number of longitudinal modes is 101, the mode interval is 300 MHz at the radiation linewidth of 30 GHz, and the length of the laser resonator is 500 mm. A Cassegrain telescope is employed to receive the lidar echo signals excited by the MLM laser pulses, which has a receiving aperture of 203 mm and a focal length of 2000 mm. A self-tuning MZI with the OPD of 1000 mm is designed as the spectral discriminator to separate aerosol Mie scattering signals and molecular Rayleigh scattering signals. A Tektronix oscilloscope is chosen for data acquisition with analog detection mode of the real-time sampling rate of 125 MS/s, resulting in the range resolution of 1.5 m in the raw data profile.
Figure 1.Schematic diagram of the multilongitudinal mode HSRL system: (a) spectrum of the MLM laser; (b) spectrum of the MLM echo signal; (c) output spectrum of the Mie channel of the MZI; (d) output spectrum of the Rayleigh channel of the MZI.
The tunable MZI is the key component in the design of the MLM-HSRL, which is composed of lenses, beam splitters (BSs), Dove prisms, a displacement system, field compensation glasses (FCGs), and photomultiplier tubes (PMTs). Lens1 collimates the MLM echo signals from the multimode fiber with a diameter of 0.8 mm, which is utilized to transfer the received backscattered light from telescope to the spectral discriminator MZI. The beam splitter BS1 divides the MLM echo signals into two parts. The reflected signals of BS1 are reflected 3 times by the Dove prisms (Prism3, Prism4, Prism5) and then reach the beam splitter BS2, which constructs the long optical path of the MZI. Field compensation glasses (FCG1, FCG2, FCG3, and FCG4) with a total length of 1165.767 mm are added to the long optical path to expand the receiving field of view of the MZI up to 5
The MZI has two channels with the complementary output signals; one channel transmits aerosol Mie scattering signals and is called the Mie channel; the other channel suppresses aerosol Mie scattering signals and is called the Rayleigh channel. The spectra of the MLM laser, lidar echo signal, and the outputs of the Mie channel and Rayleigh channel are plotted in Fig. 1 as well, to show the working principle of the MLM-HSRL. The lidar equations for both the Mie channel and the Rayleigh channel of the MLM-HSRL can be expressed as
Based on the retrieval algorithm of aerosol optical properties using the MLM-HSRL, the retrieval accuracy is highly related to the effective transmittance (
Theoretical analysis shows that the maximum value of
Figure 2.Relationship between the effective transmittance of the Mie channel for Mie scattering signals and the OPD of the MZI. (a) For the first condition of the matching degree between the MZI OPD and the laser optical cavity length; (b) for the second condition of the matching degree between the MZI OPD and laser wavelength.
Theoretical calculation shows that the temperature accuracy and pressure accuracy of the MZI and laser cavity should be controlled within
The functional diagram and the flow chart of the MLM-HSRL with a self-tuning MZI are shown in Fig. 3. The data acquisition and processing system is used not only to record the complementary outputs of the Mie channel and the Rayleigh channel, but also to connect with the proportional integral (PI) controller for the nanodisplacement platform, which can adjust the OPD of the MZI in nanometer magnitude. Theoretical simulations show that the ratio (
Figure 3.(a) Functional diagram and (b) flow chart of the MLM-HSRL with a self-tuning MZI.
Considering that the data are collected by means of analog detection mode, and the recorded data of both the Mie channel and the Rayleigh channel are the profiles related to the atmospheric information, the probing data within the detection range
3. Preliminary Experiments
After the construction of the MLM-HSRL with the self-tuning MZI, the preliminary experiments are performed in Xi’an to verify the feasibility of the MLM-HSRL and the performance of the self-tuning MZI. For quick self-tuning functionality applied in the MZI and maximum detection range of the MLM-HSRL, each profile is a summation result of 30 s (300 laser shots). Figure 4 shows the
Figure 4.PΔ distribution from the preliminary experiments by the MLM-HSRL with the fixed OPD MZI performed from 19:30 CST to 21:05 CST on March 9, 2022. (a) THI contour diagram of PΔ; (b) statistics diagram of PΔ.
Figure 5 shows the
Figure 5.PΔ distribution from the preliminary experiments by the MLM-HSRL with the dynamic OPD MZI performed from 21:30 CST to 22:55 CST on March 9, 2022. (a) THI contour diagram of PΔ; (b) statistics diagram of PΔ.
The examples of the preliminary experimental profiles and the corresponding retrieval results of aerosol optical properties from the self-tuning MLM-HSRL system are shown in Figs. 6 and 7, which were taken at a clear weather condition at 21:50 CST on March 12, 2022 (Fig. 6) and at a cloudy weather condition at 21:20 CST on April 13, 2022 (Fig. 7), respectively. In both weather conditions, the range-square-corrected signal (RSCS) data from the Rayleigh channel is smaller than the RSCS data from the Mie channel by one order of magnitude, which is due to the suppression of Mie scattering signals in the Rayleigh channel and can be explained by the values of
Figure 6.Example of the preliminary experimental data using the MLM-HSRL and the retrieval results of aerosol optical properties during a clear weather condition performed at 21:50 CST on March 12, 2022. (a) Profile of RSCS data; (b) profile of βa(r); (c) profile of αa(r); (d) profile of lidar ratio.
Figure 7.Example of the preliminary experimental data using the MLM-HSRL and the retrieved results of aerosol optical properties during a cloudy weather condition performed at 21:20 CST on April 13, 2022. (a) Profile of RSCS data; (b) profile of βa(r); (c) profile of αa(r); (d) profile of lidar ratio.
The THI diagrams of the retrieved
Figure 8.THI diagram of the retrieval results of aerosol optical properties from 21:30 CST to 22:55 CST on March 9, 2022. (a) βa(r); (b) αa(r).
4. Conclusions
The MLM-HSRL with the self-tuning MZI was constructed at the Xi’an University of Technology for aerosol measurements. Compared with the single-frequency HSRL, the MLM-HSRL technique abandons the complex techniques of laser seeding and frequency stabilization, although the MLM-HSRL technique can provide a relatively lower spectral discrimination ratio (
References
[1] M. Casazza, M. Lega, G. Liu, S. Ulgiati, T. A. Endreny. Aerosol pollution, including eroded soils, intensifies cloud growth, precipitation, and soil erosion: a review. J. Cleaner Prod., 189, 135(2018).
[2] L. Simone, M. Fabio, R. Marco, C. R. James, W. J. Ellsworth. Impact of varying lidar measurement and data processing techniques in evaluating cirrus cloud and aerosol direct radiative effects. Atmos. Meas. Tech., 11, 1639(2018).
[3] Q. Liu, X. C. Jia, J. N. Quan, J. Y. Li, X. Li, Y. X. Wu, D. Chen, Z. F. Wang, Y. G. Liu. New positive feedback mechanism between boundary layer meteorology and secondary aerosol formation during severe haze events. Sci. Rep., 8, 6095(2018).
[4] Y. Zhang, J. Tao, N. Ma, Y. Kuang, H. Su. Predicting cloud condensation nuclei number concentration based on conventional measurements of aerosol properties in the North China Plain. Sci. Total Environ., 719, 137473(2020).
[5] X. Yu, B. Zhu, M. Zhang. Seasonal variability of aerosol optical properties over Beijing. Atmos. Environ., 43, 4095(2009).
[6] V. A. Kovalev, W. E. Eichinger. Elastic Lidar(2004).
[7] S. Lolli, E. J. Welton, J. R. Campbell, E. Eloranta, S. V. Salinas. High spectral resolution lidar and MPLNET micro pulse lidar aerosol optical property retrieval intercomparison during the 2012 7-SEAS field campaign at Singapore. Proc. SPIE, 9246, 92460C(2014).
[8] J. Ren, W. Tan, X. Tian, Z. Wu, T. Zhu. Retrieval of aerosol liquid water content from high spectral resolution lidar. Sci. Total Environ., 799, 149423(2021).
[9] X. Shen, N. Wang, I. Veselovskii, D. Xiao, D. Liu. Development of ZJU high-spectral-resolution lidar for aerosol and cloud: calibration of overlap function. J. Quant. Spectrosc. Radiat. Transfer, 257, 107338(2020).
[10] S. Li, H. Di, Q. Wang, G. Han, Y. Li. Retrieval of the aerosol extinction coefficient of 1064 nm based on high-spectral-resolution lidar. J. Quant. Spectrosc. Radiat. Transfer, 256, 107298(2020).
[11] Y. Jin, N. Sugimoto, T. Nishizawa, P. Ristori, L. Otero. A concept of multi-mode high spectral resolution lidar using Mach Zehnder interferometer. EPJ Web Conf., 119, 02006(2016).
[12] P. Ristori, L. Otero, Y. Jin, N. Sugimoto, T. Nishizawa, E. Quel. Development of a high spectral resolution lidar using a multi-mode laser and a tunable interferometer. EPJ Web Conf., 119, 06005(2016).
[13] Y. Jin, N. Sugimoto, P. Ristori, T. Nishizawa, E. Quel. Measurement method of high spectral resolution lidar with a multimode laser and a scanning Mach Zehnder interferometer. Appl. Opt., 56, 5990(2017).
[14] Z. Cheng, D. Liu, Y. Zhang, C. Liu, L. Yang. Generalized high spectral resolution lidar technique with a multimode laser for aerosol remote sensing. Opt. Express, 25, 979(2017).
[15] F. Gao, H. Nan, R. Zhang, Q. Zhu, S. Stanič. Quasi-monochromatic of laser echo signals on transmittance of Mach–Zehnder interferometer for UV multi-longitudinal-mode high-spectral-resolution lidar. J. Quant. Spectrosc. Radiat. Transf., 234, 10(2019).
[16] F. Gao, T. Chen, K. J. Chen, R. Zhang, L. Wang, J. J. Liu, D. X. Hua, S. Stanič. A novel retrieval algorithm of multi-longitudinal-mode high-spectral-resolution lidar based on complex degree of coherence and the analyses of absolute errors. J. Quant. Spectrosc. Radiat. Transfer, 272, 107829(2021).
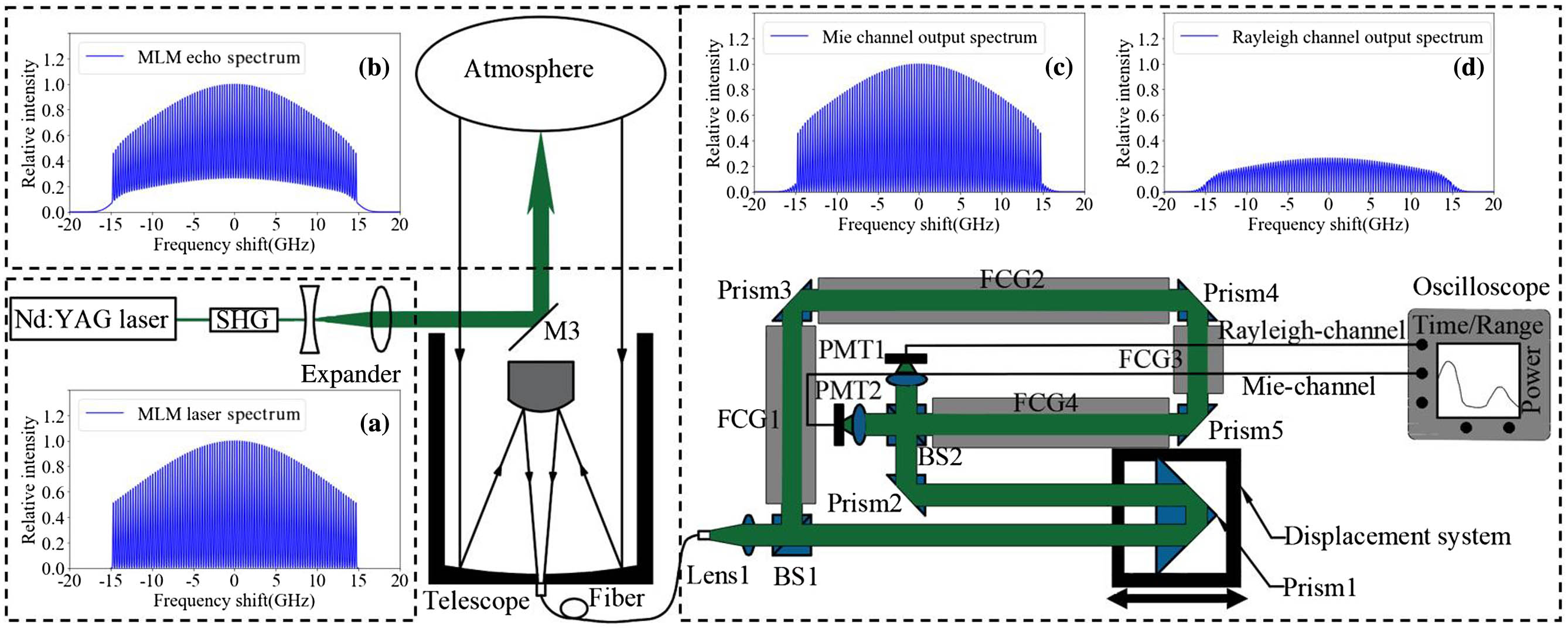
Set citation alerts for the article
Please enter your email address