Abstract
With a Nd:ScYSiO5 crystal, a high peak power electro-optically Q-switched 1.0 μm laser and tri-wavelength laser operations at the 1.3 μm band are both investigated. With a rubidium titanyle phosphate (RTP) electro-optical switcher and a polarization beam splitter, a high signal-to-noise ratio 1.0 μm laser is obtained, generating a shortest pulse width of 30 ns, a highest pulse energy of 0.765 mJ, and a maximum peak power of 25.5 kW, respectively. The laser mode at the highest laser energy level is the TEM00 mode with the M2 value in the X and Y directions to be Mx2 = 1.52 and My2 = 1.54. A tri-wavelength Nd:ScYSiO5 crystal laser at 1.3 μm is also investigated. A maximum tri-wavelength output power is 1.03 W under the absorbed pump power of 7 W, corresponding to a slope efficiency of 14.8%. The properties of the output wavelength are fully studied under different absorbed pump power.Due to excellent physicochemical and mechanical properties, oxyorthosilicate crystals have attracted great attention in the high peak power laser application. Moreover, oxyorthosilicate crystals possess a broad bandwidth resulting from the applications in tunable and ultrafast lasers. So far, the oxyorthosilicate crystals doped with different rare earth (RE) ions including [1,2], [3–5], [6,7], [8,9], and [10,11] have been well grown and applied in laser devices. In addition, as a solid solution of and , the application values of the crystal have been demonstrated by many research groups[12,13]. Due to different substitutional sites of positive ions, disordered crystals exhibit an excellent energy storage capacity and potential application in ultrafast lasers. For example, a Nd-doped crystal has a smaller emission cross section of for transition[14] and the room-temperature fluorescence lifetime was measured to be 240 μs[15]. Compared with Nd:YAG and crystals, the lower emission cross section and shorter fluorescence lifetime of the crystal imply a higher pump threshold. Nevertheless, it has a better energy storage ability and Q-switching performance. A passively Q-switched laser operating at 1.07 μm was reported in 2012[12], however, the output peak power was limited to be 0.7 kW. Compared with passive Q-switching technology using Cr4+-doped crystals or 2D materials as a saturable absorber[16–19], an electro-optical Q-switcher is a good selection to obtain a stable and high signal-to-noise ratio laser pulse. Thus, it is worth studying high peak power output characteristics of the laser based on an electro-optical Q-switcher. Furthermore, less Nd:ScYSiO5 laser research in the 1.3 μm band has been done. A high peak power 1.3 μm laser was realized when pumped by a pulsed laser diode (LD)[15]. However, the output properties, especially multiwavelength properties, need to be further investigated.
In this Letter, a high peak power, high signal-to-noise ratio electro-optically Q-switched laser at 1.0 μm and a tri-wavelength laser at 1.3 μm band are both demonstrated. The highest pulse energy, maximum peak power, and shortest pulse width are 0.765 mJ, 25.5 kW, and 30 ns, respectively. The laser mode at the highest laser energy level is the mode, with an value of and . What is more, the 1.3 μm continuous-wave (CW) tri-wavelength laser is also researched. The maximum tri-wavelength average output power is 1.03 W which is achieved under the absorbed pump power of 7 W, corresponding to a slope efficiency of 14.8%.
The experimental setups of the 1.0 μm electro-optically Q-switched laser and 1.3 μm CW laser are shown in Figs. 1(a) and 1(b), respectively. A fiber-coupled 808 nm LD with a core diameter of 400 μm and a numerical aperture (NA) of 0.22 was applied as a pump source for both laser operations. The pump beam was coupled into the laser crystal, giving a pump waist of about 400 μm. An uncoated crystal with dimensions of and a -doped concentration of 0.8 at.%. was employed as the gain medium, and the absorption efficiency of the crystal was measured at about 46%. The crystal was packed with indium foil and mounted in a water-cooled copper block. To achieve a high-quality electro-optically Q-switched laser output, a V-type folded laser cavity was selected in this experiment. The mirrors and were antireflection (AR) coated at 808 nm on the entrance face and high-transmission (HT, ) coated at 808 nm & high-reflection (HR, ) coated at 1.0 μm on the opposite face. was also AR-coated at 808 nm on the entrance face but HT-coated at 808 nm & HR-coated at 1.3 μm on the opposite face. The radii of curvature for the three mirrors are , 200 mm, and 200 mm, respectively. The output mirrors and were plane mirrors with transmissions of at 1.0 μm and , 3%, and 8% at 1.3 μm, respectively. A polarization beam splitter (PBS) was inserted into the laser cavity to realize a high signal-to-noise ratio linearly-polarized laser output. The average output power was measured by a laser power meter (Fieldmax-II, Coherent). The temporal pulse profile was recorded with a digital oscilloscope (Rohde & Schwarz, RTO2012, 1 GHz) together with a fast InGaAs photon detector (New Focus, model 1611). The laser spectra were measured by an optical spectrum analyzer (Yokogawa, AQ6370C).
Sign up for Chinese Optics Letters TOC. Get the latest issue of Chinese Optics Letters delivered right to you!Sign up now
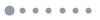
Figure 1.(a) Experimental configuration of the 1.0 μm electro-optically Q-switched laser. (b) Experimental configuration of the 1.3 μm CW laser.
In this experiment, a V-type cavity is applied to achieve 1.07 μm Q-switched laser operation of the crystal by employing an RTP electro-optical Q-switcher, a PBS, and a quarter-wave plate. According to previous research[13], the polarized relationship of the dual-wavelength laser is orthogonal polarization. Thus, due to polarization loss, insertion loss of the PBS and plate, the output power and efficiency of the electro-optically Q-switched laser are limited. Figure 2 shows the variations of the average output power with the absorbed pump power at different repetition rates. As shown in Fig. 2, the output power increases with the increase of the absorbed pump power at the repetition frequencies of both 667 Hz and 12 kHz. The maximum output power of the electro-optically Q-switched laser is obtained to be 0.71 W at a repetition rate of 12 kHz. With a spectrum analyzer, the electro-optically Q-switched laser spectrum was recorded under the maximum pump power, which is presented in the inset of Fig. 2. The laser spectrum is centered at a single wavelength of 1074.8 nm.
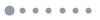
Figure 2.Electro-optically Q-switched laser output power versus absorbed pump power. Inset: the laser spectrum of the electro-optically Q-switched laser.
The dependence of the electro-optically Q-switched laser pulse width at the repetition frequencies of 667 Hz and 12 kHz on the absorbed pump power is depicted in Fig. 3. The pulse width decreases with the improvement of the absorbed pump power for both different repetition rates of the Q-switcher. The shortest pulse widths of 30 ns and 52 ns are obtained under the pulse repetition rates of 667 Hz and 12 kHz, respectively. The corresponding highest single pulse energy and peak power of the Q-switched laser are calculated to be 0.765 mJ and 25.5 kW, respectively, at the pulse frequency of 12 kHz. The single-pulse profile and pulse train of the electro-optically Q-switched laser are shown in Fig. 4. We can observe that no satellite is present before or after the pulse and the time jitter from pulse to pulse is minimal, indicating that the Q-switched laser is stable. The laser beam quality (M2) was also investigated at the highest output power level by using the knife edge technique. Figure 5(a) demonstrates the variation of the laser beam size with the different positions. By fitting the data to express the factor M2, we can estimate that the values in the X and Y directions are about 1.52 and 1.54, respectively. The corresponding 2D and 3D beam spatial distributions of the Q-switched laser were measured with a pyroelectric infrared camera and displayed in Figs. 5(b) and 5(c), respectively. As can be seen, the transverse mode presents a perfect Gaussian profile, indicating that the Q-switched laser is in the mode.
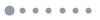
Figure 3.Laser pulse width versus the absorbed pump power.
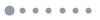
Figure 4.Typical electro-optically Q-switched laser pulse trains. (Inset: the single laser pulse profile.)
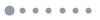
Figure 5.M2 value and laser beam profile at the highest output power.
Table 1 summarizes all the Q-switched results achieved with different Nd-doped oxyorthosilicate crystals, such as , , , and . A. Power, P. Width, P. Energy, and P. Power listed in the Table 1 mean average power, pulse width, pulse energy, and peak power, respectively. Compared with previous experimental results obtained by Nd-doped oxyorthosilicate crystals, our results demonstrate a higher single-pulse energy of 765 μJ and a peak power of 25.5 kW. Compared with Cr:YAG as a passive Q-switcher, the RTP EO Q-switcher could be an excellent selection to obtain a high peak power laser with a high signal-to-noise ratio and high stability.
Materials | Q-switcher | A. Power (W) | P. Width (ns) | P. Energy (μJ) | P. Power (kW) | Refs. |
---|
Nd:ScYSiO5 | Cr:YAG | 1.03 | 24 | 16.2 | 0.7 | [12] |
Nd:LuYSiO5 | Cr:YAG | −0.27 | 29 | 34.3 | 1.2 | [20] |
Nd:GdYSiO5 | Cr:YAG | 1.20 | 87.8 | 300 | 3.4 | [21] |
Nd:Lu2SiO5 | Cr:YAG | −0.47 | 12.4 | 29 | 2.3 | [22] |
Nd:Sc2SiO5 | Cr:YAG | −0.13 | 27 | 44.8 | 1.7 | [23] |
Nd:ScYSiO5 | RTP EO Q-switcher | 0.51 | 30 | 765 | 25.5 | This work |
Table 1. Comparison of the Q-switched laser properties with different Nd-doped oxyorthosilicate crystals
As shown in Fig. 1(b), a 1.3 μm CW multiwavelength laser operation was carried out by tuning the laser cavity. The length of the linear cavity was about 22 mm. The CW laser output characteristics were fully demonstrated by using several different output mirrors, as the detailed experimental data show in Fig. 6. The threshold is only 0.024 W, with the output coupler indicating that the crystal possesses a good optical quality. With the output coupler of , the average output power increases almost linearly with the increase of the pump power. The maximum average output power of 1.03 W is generated under the absorbed pump power of 7 W with the transmission of , giving a slope efficiency of 14.8%. Due to the thermal effect of the crystal, the output efficiency decreased when the absorbed pump power was more than 7 W.
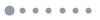
Figure 6.Average output power versus absorbed pump power of the 1.3 μm CW laser.
During the 1.3 μm experiment, the laser spectral properties were also studied and we found that the intensity ratio of the various oscillating modes can be tuned by adjusting the pump power or employing output couplers with different transmissions. As shown in Fig. 7, with the improvement of pump power, various output wavelengths have been achieved by the optical spectrum analyzer. The initial oscillated wavelength is a single wavelength at 1358.7 nm with the transmission of , while for the the initial oscillated wavelength is also a single wavelength at 1358.6 nm. With the improvement of the pump power, gain competition between various wavelengths appeared, and the phenomenon of simultaneous multiwavelength oscillation was obtained at the higher pump power level. The tri-wavelength laser spectra with different transmissions of and have been presented in Figs. 7(b) and 7(d), respectively. With the output coupler of , the tri-wavelength simultaneous oscillation appeared under the absorbed pump power of 3.9 W, while that is 6.4 W for the output coupler of .
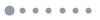
Figure 7.Output spectra of the 1.3 μm multiwavelength laser.
In conclusion, the laser output characteristics of the disordered crystal have been studied both for and operations. A stable and high signal-to-noise ratio 1.0 μm laser with high peak power is obtained by selecting an RTP electro-optical switcher as the optical modulator. The achieved shortest pulse width, maximum peak power, and highest pulse energy are 30 ns, 25.5 kW, and 765 μJ, respectively. At the highest laser output power, the laser mode is the mode with M2 values in the X and Y directions of about 1.52 and 1.54, respectively. Besides taking advantage of the broad bandwidth gain, the 1.3 μm tri-wavelength CW laser is investigated in detail with different output couplers. The maximum tri-wavelength average output power is 1.03 W with the output coupler of , corresponding to a slope efficiency of 14.8%. The tri-wavelength is measured to be 1348.5 nm, 1358.8 nm, and 1363.0 nm for output coupler; and 1358.2 nm, 1362.8 nm, and 1386.7 nm for output coupler, respectively.
References
[1] L. H. Zheng, J. Xu, L. B. Su, H. J. Li, Q. G. Wang, W. Ryba-Romanowski, R. Lisiecki, F. Wu. Opt. Lett., 34, 3481(2009).
[2] M. Li, L. F. Wang, S. Han, C. L. Yu, D. P. Chen, W. Chen, L. L. Hu. Chin. Opt. Lett., 16, 080601(2018).
[3] M. Jacquemet, C. Jacquemet, N. Janel, F. Druon, F. Balembois, P. Georges, J. Petit, B. Viana, D. Vivien, B. Ferrand. Appl. Phys. B, 80, 171(2015).
[4] B. K. Brickeen, E. Geathers. Opt. Express, 17, 8461(2009).
[5] Y. D. Wang, K. J. Yang, S. Z. Zhao, M. Li, T. Li, W. C. Qiao, S. D. Liu, X. C. Su, B. T. Zhang, J. L. He, L. H. Zheng, L. B. Su, J. Xu. Opt. Eng., 57, 066101(2018).
[6] T. Schweizer, T. Jensen, E. Heumann, G. Huber. Opt. Commun., 118, 557(1995).
[7] X. Fernandez-Gonzalvo, Y. H. Chen, C. M. Yin, S. Rogge, J. J. Longdell. Phys. Rev. A, 92, 062313(2015).
[8] L. H. Zheng, J. Xu, L. B. Su, H. J. Li, W. Ryba-Romanowski, R. Lisiecki, P. Solarz. Appl. Phys. Lett., 96, 121908(2010).
[9] T. L. Feng, S. Z. Zhao, K. J. Yang, G. Q. Li, D. C. Li, J. Zhao, W. C. Qiao, J. Hou, Y. Yang, J. L. He, L. H. Zheng, Q. G. Wang, X. D. Xu, L. B. Su, J. Xu. Opt. Express, 21, 24665(2013).
[10] B. Q. Yao, Z. P. Yu, X. M. Duan, Z. M. Jiang, Y. J. Zhang, Y. Z. Wang, G. J. Zhao. Opt. Express, 17, 12582(2009).
[11] X. M. Duan, C. P. Qian, Y. J. Shen, L. B. Su, L. H. Zheng, L. J. Li, B. Q. Yao, T. Y. Dai. Opt. Express, 27, 4522(2019).
[12] S. D. Liu, L. H. Zheng, J. L. He, J. Xu, X. D. Xu, L. B. Su, K. J. Yang, B. T. Zhang, R. H. Wang, X. M. Liu. Opt. Express, 20, 22448(2012).
[13] V. Aleksandrov, H. Iliev, A. Trifonov, L. Zheng, J. Xu, L. Su, I. Buchvarov. CLEO, JTu4A.127.
[14] S. D. Liu, L. H. Zheng, J. Xu, Y. P. Zhang, H. Y. Zhang, D. H. Li, T. Q. Ren, B. T. Zhang, J. L. He. Chin. Opt. Lett., 14, 021406(2016).
[15] L. Li, M. Y. Cao, S. D. Liu, Y. D. Peng, L. H. Zheng, J. Xu. Optik, 140, 248(2017).
[16] S. D. Liu, Q. G. Wang, K. Wang, Y. P. Yao, H. Y. Zhang, T. Q. Ren, Z. M. Yin, F. L. Du, B. T. Zhang, J. L. He. Opt. Lett., 42, 3972(2017).
[17] Y. P. Yao, N. Cui, Q. G. Wang, L. L. Dong, S. D. Liu, D. L. Sun, H. Y. Zhang, D. H. Li, B. T. Zhang, J. L. He. Opt. Lett., 44, 2839(2019).
[18] Y. P. Yao, X. W. Li, R. G. Song, N. Cui, S. D. Liu, H. Y. Zhang, D. H. Li, Q. G. Wang, Y. Xu, J. L. He. RSC Adv., 9, 14417(2019).
[19] B. Guo. Chin. Opt. Lett., 16, 020004(2018).
[20] S. D. Zhuang, X. D. Xu, Z. P. Wang, D. Z. Li, H. H. Yu, J. Xu, L. Guo, L. J. Chen, Y. G. Zhao, X. G. Xu. Laser Phys, 21, 684(2011).
[21] C. Feng, Z. J. Liu, Z. H. Cong, H. B. Shen, Y. F. Li, Q. P. Wang, J. X. Fang, X. D. Xu, X. Y. Zhang. Laser Phys. Lett., 12, 125806(2015).
[22] X. D. Xu, J. Q. Di, J. Zhang, D. Y. Tang, J. Xu. Opt. Mater., 51, 241(2016).
[23] K. J. Yang, S. Z. Zhao, G. Zhang, K. Cheng, G. Q. Li, D. C. Li, J. L. Xu, J. L. He, L. H. Zheng, F. Wu, Q. G. Wang, L. B. Su, J. Xu. Laser Phys. Lett., 9, 10(2012).