Abstract
We report on a spectral beam combination of five narrow-linewidth fiber amplifiers. The five-channel output beams are combined in both the near and far field using a polarization-independent diffraction grating that mainly preserves the beam quality of the individual amplifiers. Each amplifier contains a two-stage preamplifier and a main amplifier delivering about 240 W of optical power, which allows a total combined output power of 1.23 kW with an efficiency of over 95%.Yb-doped fiber lasers have been regarded as a charming laser system recently in the material processing area owing to their unique properties such as high optical efficiency, high power capability, compact and robust design, and high beam quality. The power of Yb-doped fiber laser has risen rapidly in the past ten years[1]. Scaling up the output power of a single-fiber laser to a higher power level faces significant challenges such as nonlinear effects, thermal loading, and fiber damage[2–4]. Beam combining techniques have been proposed to achieve higher powered fiber lasers that can scale up the output power by combining multiple lasers and meanwhile maintain a good beam quality[5,6]. Typically the beam combining techniques can be classified into two categories: coherent beam combining and spectral beam combining (SBC). The coherent beam combining method is strict with the elementary beam on polarization, phase, and linewidth, which makes it tough to develop to a higher power level[7–9]. The SBC method, which is the topic of this Letter, has no restriction on the phase and polarization of the incident beams, thus becoming a promising and reliable approach to achieving extremely high-power operation[10–15]. In this case, a dispersive beam-combining element such as a diffraction grating is used to merge the outputs from multiple, spectrally distinct lasers into a single diffraction-limited beam.
As is well known, there are two ways to obtain a high-power output for a beam-combining method. Increasing the power of the single channel fiber laser can be seen as one way to boost the combining output power, while adding the incident channels is the other one. The SBC method has the scaling potential owing to its inherent advantages: the relatively relaxed requirement against the coherent method on the individual channel linewidth of tens of gigahertz and the wide wavelength span within the entire gain bandwidth. A lot of effort has been made to boost the output power of the SBC. Loftus et al. reported a spectrally beam-combined 1-μm fiber laser that produces 522 W of average power with a near-diffraction-limited beam quality[11]. Wirth et al. achieved 2 kW of combined output power using a polarization-independent dielectric reflective diffraction grating to merge four channels of fiber lasers together[14]. Two years later, they scaled up their previous result to 8.2 kW by boosting the power of the individual incident fiber lasers from 500 W to 2 kW[15].
In this contribution, we demonstrate a SBC of five narrow-linewidth fiber amplifiers emitting around 1068.4 nm wavelength that are seeded by super fluorescent sources. Each amplifier contains a two-stage preamplifier and a main amplifier delivering about 240 W optical power. The five-channel output beams are combined in both near and far field for a total power of 1.23 kW using a polarization-independent diffraction grating with a diffraction efficiency of 95.6%, which mainly preserves the beam quality of the individual amplifiers. To the best of our knowledge, this is the highest combined power of super fluorescent sources.
Sign up for Chinese Optics Letters TOC. Get the latest issue of Chinese Optics Letters delivered right to you!Sign up now
The optical layout for the five-channel SBC fiber lasers is illustrated in Fig. 1. The seed sources of the five individual elementary lasers are all super fluorescent sources that are obtained by an all-fiber broadband-amplified spontaneous-emission (ASE) source and a linewidth-narrowing module. The seed sources are characterized by a 70-pm linewidth and 60 mW optical power. The five distinct wavelengths are achieved by five different pairs of fiber Bragg gratings with the corresponding wavelengths of 1055.7, 1058.5, 1064.8, 1071.9, and 1081.0 nm.
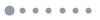
Figure 1.Optical layout for the five-channel SBC fiber lasers. Five beams are combined by a diffraction grating with a density of . The wavelength-dependent incidence angles can be aligned by means of the steering mirrors. YDFA is the Yb-doped fiber amplifier; CCD is the charged coupled device.
The seed sources are first boosted by a two-stage Yb-doped preamplifier from 60 mW to 70 W. Stage 1 is based on a 4-m large-mode-area Yb-doped double-cladding fiber (PLMA-YDF-10/125-VIII, Nufern) pumped by a 10 W 976-nm laser diode through a combiner. Stage 2 is based on a 5-m PLMA-YDF-10/125-VIII fiber pumped by six 15 W 976-nm laser diodes through a combiner. Each stage is followed by an all-fiber pump stripper to remove the residual pump light. An isolator is used between stage 1 and stage 2 for the purpose of protecting the preamplifier from backscattering light. Then a main amplifier boosts the power to . The main amplifier is based on a 10-m large-mode-area Yb-doped double-cladding fiber (LMA-YDF-20/400, Nufern) for high-power operation that is pumped by a 400 W 976-nm laser diode. This active fiber is placed in an aluminum water-cooled heatsink and coiled properly to introduce losses for the high-order modes. The output end of the main amplifier is connected to a fiber optic cable (QBH, Optoskand). The cable contains 15 m of Nufern LMA-GDF-20/400-M delivery fiber, which is coiled properly to introduce losses for the high-order modes. The diameter of the laser beam output from the collimator is 12 mm, corresponding to the 0.06 NA of the delivery fiber and 100-mm focal length of the collimator.
The five beamlets emitting from the collimators are combined by a multilayer dielectric reflective diffraction grating. The grating is optimized for both TE and TM polarization in a wavelength range from 1040 to 1090 nm. Thus, no polarization control of the main amplifier is required. The grating has a period of 1040 nm ( line density). The grating is aligned in a first-order Littrow configuration at an angle of 30.85°, corresponding to the 1068.4-nm wavelength in the horizontal plane (the grating dispersive plane) and tipped 1.5° in the vertical direction. The wavelength-dependent angle of incidence can be adjusted by means of a group of steering mirrors (HR 1050–1090 nm at 45°); thus the beamlets of the five channels are geometrically overlapped in both the near and far field. The distance between the grating and steering mirrors is about 4 m, which is enough for the alignment of the optical elements. The combined output beam is separated into two parts by a beam splitter, which removes about 0.1% () of the combined beam for the beam quality measurement and leaves the rest of the 99.9% for the power monitor. Images acquired with a charge coupled device (CCD) camera are used to match the divergence angles for the individual channels and spatially overlap the beams on the grating and in the far field.
The optical spectrum of the combined beam is depicted in Fig. 2. The spectral composition of the combined beam is measured with an optical spectrum analyzer (YOKOGAWA AQ6370B) with a resolution of 20 pm, corresponding to 247 W at 1055.7 nm, 273 W at 1058.5 nm, 263 W at 1064.8 nm, 218 W at 1071.9 nm, and 229 W at 1081.0 nm. In this Letter, the channel to channel wavelength intervals are arbitrarily chosen to provide ease of alignment with standard mirror sizes. The smallest wavelength interval in this experiment is 2.8 nm, implying that more channels within the gain bandwidth can be added into this experimental system, thus achieving a higher combining power. The ASE background is suppressed by a factor of .
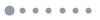
Figure 2.Spectrum of the combined beam. The smallest wavelength interval is 2.8 nm between 1055.7 and 1058.5 nm.
The power-combining efficiency for the SBC system, defined as the sum of the individual channel power prior to the steering mirrors divided by the combined-beam power, is 95.6%. The far-field pattern of the combined output beam is shown in Fig. 3. It can be seen that the combined beam of the five channels mainly preserves the good quality of the incident beam. However, we find that the horizontal diameter becomes a little larger than the original beam while the vertical diameter remains unchanged. This phenomenon can be attributed to two reasons: the finite linewidth of our incident beam and the slight misalignment of the incident beams. According to the theory of the diffraction grating, dispersion will be introduced to the diffracted beam in the dispersal plane when the incident beam has a finite linewidth. On the other hand, this experiment is conducted in a noisy environment because many cooling machines are used in this system. Inevitably some vibration is introduced to the optical platform, thus making the output beam shake. Hence, the beam quality will degrade to some extent.
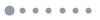
Figure 3.Far-field pattern of the combined output beam, mainly maintaining the good beam quality of the incident beams.
In conclusion, we achieve SBC of five fiber amplifiers that are all seeded by the ASE source. The five beams are combined by using a polarization-independent diffraction grating with a density of . The total power of the combined output beam is up to 1.23 kW, with a combining efficiency of about 95%. The image acquired by the CCD shows that the horizontal size of the output beam becomes a little larger than the original one while the vertical size remains same. This is attributed to the finite linewidth of the incident beam. Some methods need to be employed to reduce the linewidth, thus improving the beam quality of the combined beam. Further power scaling of this system can be realized by increasing the power of individual fiber amplifiers and adding the elementary channels.
References
[1] J. Limpert, F. Röser, S. Klingebiel, T. Schreiber, C. Wirth, T. Peschel, R. Eberhardt, A. Tünnermann. IEEE J. Sel. Topics Quantum Electron., 13, 537(2007).
[2] I. Dajani, C. Zeringue, C. Lu, C. Vergien, L. Henry, C. Robin. Opt. Lett., 35, 3114(2010).
[3] V. R. Suradeepa. Opt. Express, 21, 4677(2013).
[4] A. Flores, I. Dajani, D. Hult, C. Robin. Proc. SPIE, 8964, 89640R(2014).
[5] T. Y. Fan. IEEE J. Sel. Topics Quantum Electron., 11, 567(2005).
[6] S. J. Augst, J. K. Ranka, T. Y. Fan, A. Sanchez. J. Opt. Soc. Am. B, 24, 1707(2007).
[7] S. M. Redmond, D. J. Ripin, C. X. Yu, S. J. Augst, T. Y. Fan, P. A. Thielen, J. E. Rothenberg, G. D. Goodno. Opt. Lett., 37, 2832(2012).
[8] P. A. Thielen, J. G. Ho, D. A. Burchman, G. D. Goodno, J. E. Rothenberg, M. G. Wickham, A. Flores, C. A. Lu, B. Pulford, C. Robin, A. D. Sanchez, D. Hult, K. B. Rowland. Opt. Lett., 37, 3741(2012).
[9] Y. Yang, M. Hu, B. He, J. Zhou, H. Liu, S. Dai, Y. Wei, Q. Lou. Opt. Lett., 38, 854(2013).
[10] T. H. Loftus, A. Liu, P. R. Hoffman, A. Thomas, M. Norsen, C. E. Hamilton, E. Honea. Proc. SPIE, 6102, 61020S(2006).
[11] T. H. Loftus, A. Liu, P. R. Hoffman, A. M. Thomas, M. Norsen, R. Royse, E. Honea. Opt. Lett., 32, 349(2007).
[12] T. H. Loftus, A. M. Thomas, P. R. Hoffman, M. Norsen, R. Royse, A. Liu, E. C. Honea. IEEE J. Sel. Topics Quantum Electron., 13, 487(2007).
[13] O. Schmidt, C. Wirth, I. Tsybin, T. Schreiber, R. Eberhardt, J. Limpert, A. Tünnermann. Opt. Lett., 34, 1567(2009).
[14] C. Wirth, O. Schmidt, I. Tsybin, T. Schreiber, T. Peschel, F. Brückner, T. Clausnitzer, J. Limpert, R. Eberhardt, A. Tünnermann, M. Gowin, E. ten Have, K. Ludewigt, M. Jung. Opt. Express, 17, 1178(2009).
[15] C. Wirth, O. Schmidt, I. Tsybin, T. Schreiber, R. Eberhardt, J. Limpert, A. Tünnermann, K. Ludewigt, M. Gowin, E. Have, M. Jung. Opt. Lett., 36, 3118(2011).