
- Chinese Optics Letters
- Vol. 20, Issue 6, 061402 (2022)
Abstract
1. Introduction
High-power, high-brightness semiconductor lasers have a series of advantages of small size, light weight, high conversion efficiency, long life-time, direct modulation, and easy integration with other semiconductor devices. They are widely used in medical treatment, pumping of solid-state and fiber lasers, industrial processing, optical communications, and so on[
Since Daneu et al. demonstrated the technique of SBC using a laser diode array at 2.05 µm, obtaining 1.8 W with 50% SBC efficiency (ratio of SBC output power to free run power)[
Compared with other beam combining methods, SBC must provide enough feedback in the setup to lock each emitter to the different wavelength so that the output coupler generally has a minimum reflectivity of about 10%. This limits the SBC efficiency and the further increase in output power. In addition, during the packaging process of the laser array, due to the mismatch of the thermal expansion coefficient between the chip and the heat sink, the thermal stress causes the beam of each emitter of the laser array not to be in a straight line. This shows the inevitable existence of the smile effect. Since the dimension of the light-emitting area perpendicular to the PN junction is in the order of micrometers, a small displacement will have a great impact on the beam quality of the diode laser. It will also bring greater difficulty to subsequent beam shaping and feedback effect of the external cavity. Based on the characteristics of the broad-area laser array, the waveguide in the vertical direction is narrower than in the horizontal direction, so the beam feedback of the vertical direction is relatively more difficult. If the packaging is further affected by the smile effect, the feedback will be more difficult to predict. We believe that the SBC efficiency can be further improved if the effective feedback of the external cavity beam can be increased.
Sign up for Chinese Optics Letters TOC. Get the latest issue of Chinese Optics Letters delivered right to you!Sign up now
Considering these problems, we tried to adopt a thicker waveguide, where the feedback light from the external cavity is easier to return to the internal cavity for gain oscillation, and it may be possible to make the SBC efficiency close to 100% or even higher.
2. Design and Simulations
The simulated optical path diagram is shown in Fig. 1, including a detector, a laser, a fast-axis collimation lens (FAC), a slow-axis collimation lens (SAC), a transform lens, a transmission grating, and a reflector. Furthermore, to weaken the effect of the smile effect on external cavity locking, we used the beam shaping element, that is, the beam transformation system (BTS). It is composed of a diagonal lens array and can rotate the beam of each emitter of the laser array, so that the slow-axis optical field distribution becomes vertical, and the fast-axis optical field distribution becomes horizontal. When the external cavity beams are combined, the original vertical smile effect can be converted into a difference in horizontal position.
Figure 1.Simulated optical path diagram. FAC, fast-axis collimation lens; BTS, beam transformation system; SAC, slow-axis collimation lens.
We simulated the setup by using the method of ray tracing. The laser beam with a certain power passes through the entire setup and reaches the reflector. Then, the beam is reflected and passes through the setup again. We use the green light to represent the light emitted by the laser source and the red light to represent the reflected light, and the arrows indicate the direction of beam propagation. We place a detector behind the source. We use the reflected light detected by the detector to qualitatively analyze the influence of the waveguide thickness of the external cavity. The feedback efficiency (FE) is defined by the ratio of the power detected by the detector to the power of the laser source itself. Note that the FE mentioned in this section and the SBC efficiency are not the same.
Because of the principle of SBC, the center wavelength of each emitter of the laser array and the incident angle relative to the grating satisfy the grating equation,
In addition, the broadening of spectral width corresponding to diverse focal lengths of the transform lens is different, and the degree of grating dispersion is also different. In order to consider that the line-width will be affected by the transform lens and the beam feedback will be affected by the grating dispersion, we set three wavelengths for the light source. They are the central wavelength and the wavelengths at half-maximum, respectively. The power of the center wavelength is twice the power of the wavelengths at half-maximum.
We set the light source to several lasers with different waveguide thicknesses, such as 1.5 µm, 2.5 µm, 3.5 µm, 4.5 µm, and 5.5 µm, respectively. The fast-axis beam was collimated using the FAC with a focal length of 365 µm, and then the beam was rotated 90° by the BTS. The slow-axis beam was collimated by the SAC with a focal length of 50 mm. The effective focal lengths of the transform lens are from 100 mm to 500 mm, with an interval of 50 mm. The transmission grating was with 1850 lines/mm, and first-order diffraction efficiency was above 90% at a wavelength at 970 nm.
From Fig. 2, for a laser with a fixed waveguide thickness, the FE increases slightly and then decreases significantly as the focal length of the transform lens increases. The reason is that if the focal length of the transform lens is small, its collimation effect is not good enough, and the spectral width is large. It will increase the dispersion of the grating. If the focal length becomes larger, the optical path of the entire SBC structure becomes longer. The feedback effect of the beam will be weakened. Taking the waveguide thickness of 1.5 µm as an example, when the focal length is 100 mm, the FE is 35.13%; when the focal length increases to 150 mm, the FE increases to 36.33%; and then the FE drops to 26.16% when the focal length increases to 500 mm.
Figure 2.Characteristics of the FE and focal length of the transform lens with five different waveguide thicknesses.
Also, we can see that the FE is positively correlated with the thickness of the waveguide. The thicker the waveguide is, the higher the FE is. Taking the focal length of 100 mm as an example, the FE of the lasers corresponding to the five waveguide thicknesses is 35.13%, 42.85%, 45.35%, 46.36%, and 47.58% in order. When the focal length is increased to 500 mm, the FE becomes 26.16%, 34.33%, 38.71%, 41.74%, and 45.54% in turn. Through the simulation results, it can also be found that for a laser with a thicker waveguide, the FE will decrease relatively slowly as the focal length of the transform lens increases.
Some applications have requirements for spectral width. For example, some applications hope to achieve higher power and higher brightness through as many laser arrays as possible. The spectral width
3. Experimental Results and Discussion
We have illustrated that laser diodes with thicker waveguides have several distinct advantages for external cavity feedback, so we used this kind of laser to perform the SBC experiment. Based on the simulation results and the optimization of the laser chip, the laser array we used in the experiment was a large optical cavity (LOC) laser with a waveguide thickness of 5.2 µm[
In the experimental setup shown in Fig. 3, the 970 nm LOC laser array is composed of 19 elements, each around 100 µm wide with a pitch of 500 µm. The cavity length of the laser array is 2 mm. The front facet of the laser array was plated with an anti-reflection coating of
Figure 3.Schematic diagram of the SBC setup. (a) Top view; (b) side view.
Figure 4 shows the free run power, SBC power, and SBC efficiency as a function of the driving current. The laser array worked at the chilling temperature of 20°C and CW operating mode. At small driving currents, the SBC power is higher than the free run power. The reason is that SBC can increase the gain oscillation due to the feedback of the external cavity, so the threshold current can be reduced. Therefore, the SBC efficiency may exceed 100%. The maximum electrical to optical conversion efficiency is 42% at 50 A. Under high driving currents, the curve between the SBC power and the current is still linear, and there is no sign that thermal rollover is about to occur. At 90 A, the SBC output power is 59.2 W, and the SBC efficiency is 102.8%. This is mainly because the mode selection element is inserted into the external cavity of SBC, the beam quality is better than when the beams are not combined. The number of modes is smaller, the gain of the fundamental mode becomes larger, and the ratio of the fundamental mode is higher. Overall, the use of the LOC laser can increase the FE, thereby improving the SBC efficiency. It can break through the current bottleneck when the SBC efficiency cannot exceed 100% under high current.
Figure 4.SBC efficiency, SBC power, and free run power with the change of current.
The measured spectrum behind the output coupler is shown in Fig. 5. It can be seen that all 19 laser emitters have achieved spectral locking even at a high driving current of 90 A. The intensity of the few peaks on the left is relatively low compared to the other peaks, which may be due to the problem of laser array packaging that causes the intensity of the emitters corresponding to these peaks to be affected. The measured spectral width
Figure 5.Measured spectrum of SBC output.
We use the beam parameter product (BPP) for evaluating beam quality. The BPP of the combined beam of a specific wavelength is determined by the near-field spot size and far-field divergence. We measured the beam quality by an objective lens with a focal length of 250 mm. The near-field spot size of the beam is
4. Conclusion
In summary, we have demonstrated a structure of SBC based on the 970 nm LOC laser array. We achieve the spectral locking of each emitter and obtain the output beam with the overlap of the near-field and far-field spots finally. As a result, a CW power of 59.2 W, SBC efficiency of 102.8%, and spectral width of 11.67 nm were obtained. The BPP is about 1.10 times that of a single emitter in the horizontal direction and 1.29 times that of a single emitter in the vertical direction. The most prominent advantage of the LOC laser is its thicker waveguide. It can not only reduce the power density so that the optical mirror is not easily damaged, but also make the external cavity beam easier be fed back to improve the SBC efficiency and make the SBC efficiency as unaffected as possible when the focal length of the transform lens changes.
References
[1] M. Hemenway, Z. Chen, W. Urbanek, D. Dawson, L. Bao, M. Kanskar, M. DeVito, R. Martinsen. Continued advances in high brightness fiber-coupled laser modules for efficient pumping of fiber and solid-state lasers. Proc. SPIE, 10514, 105140P(2018).
[2] M. Kelemen, J. Gilly, P. Friedmann, S. Hilzensauer, L. Ogrodowski, H. Kissel, J. Biesenbach. Diode lasers optimized in brightness for fiber laser pumping. Proc. SPIE, 10514, 105140F(2018).
[3] L. Li. The advances and characteristics of high-power diode laser materials processing. Opt. Laser Eng., 34, 231(2000).
[4] F. Bachmann, R. Poprawe, P. Loosen. High Power Diode Lasers(2007).
[5] C. Cook, T. Fan. Spectral beam combining of Yb-doped fiber lasers in an external cavity. Advanced Solid State Lasers, PD5(1999).
[6] V. Daneu, A. Sanchez, T. Y. Fan, H. K. Choi, G. W. Turner, C. C. Cook. Spectral beam combining of a broad-stripe diode laser array in an external cavity. Opt. Lett., 25, 405(2000).
[7] C. Hamilton, S. Tidwell, D. Meekhof, J. Seamans, N. Gitkind, D. Lowenthal. High power laser source with spectrally beam combined diode laser bars. Proc. SPIE, 5336, P1(2004).
[8] P. Crump, S. Knigge, A. Maaßdorf, F. Bugge, S. Hengesbach, U. Witte, H. D. Hoffmann, B. Köhler, R. Hubrich, H. Kissel, J. Biesenbach, G. Erbert, G. Traenkle. Low-loss, smile-insensitive external frequency-stabilization of high power diode lasers enabled by vertical designs with extremely low divergence angle and high efficiency. Proc. SPIE, 8605, 86050T(2013).
[9] A. L. Glebov, P. O. Leisher, R. K. Huang, B. Chann, J. Burgess, B. Lochman, W. Zhou, M. Cruz, R. Cook, D. Dugmore. Teradiode’s high brightness semiconductor lasers. Proc. SPIE, 9730, 97300C(2016).
[10] J. Zhang, H. Peng, X. Fu, Y. Liu, L. Qin, G. Miao, L. Wang. CW 50W/M2=10.9 diode laser source by spectral beam combining based on a transmission grating. Opt. Express, 21, 3627(2013).
[11] Z. Zhu, L. Gou, M. Jiang, Y. Hui, H. Lei, Q. Li. High beam quality in two directions and high efficiency output of a diode laser array by spectral-beam-combining. Opt. Express, 22, 17804(2014).
[12] B. Wang, W. Guo, Z. Guo, X. Dan, X. Chen. Spectral beam combining of multi-single emitters. Proc. SPIE, 9733, 97330F(2016).
[13] S. Sun, H. Tan, H. Meng, L. Guo, S. Gao, D. Wu, F. Xu. Coupler free grating external cavity spectral beam combining of diode laser stacks. Chin. J. Lasers, 45, 1001007(2018).
[14] X. Lin, G. Lin, P. Zhao, L. Wang, H. Yu. Generation of high brightness diode laser by using spectral and polarization beam combination. Opt. Laser Technol., 116, 219(2019).
[15] Y. Xu, Z. Wang, F. Zhang, R. Yang, X. Liu, Y. Song, Y. Bo, Q. Peng, Z. Xu. High-efficiency spectral-beam-combined 930 nm diode laser source. Chin. Phys. Lett., 37, 054203(2020).
[16] S. Zhan, Z. Wu, F. He, J. Zhang, J. You, Y. Ma. Influence of transform-lens focal length on spectral beam combining in an external cavity with a microlens array. Opt. Commun., 387, 223(2017).
[17] S. Zhao, A. Qi, M. Wang, H. Qu, Y. Lin, F. Dong, W. Zheng. High-power high-brightness 980 nm lasers with <50% wall-plug efficiency based on asymmetric super large optical cavity. Opt. Express, 26, 3518(2018).
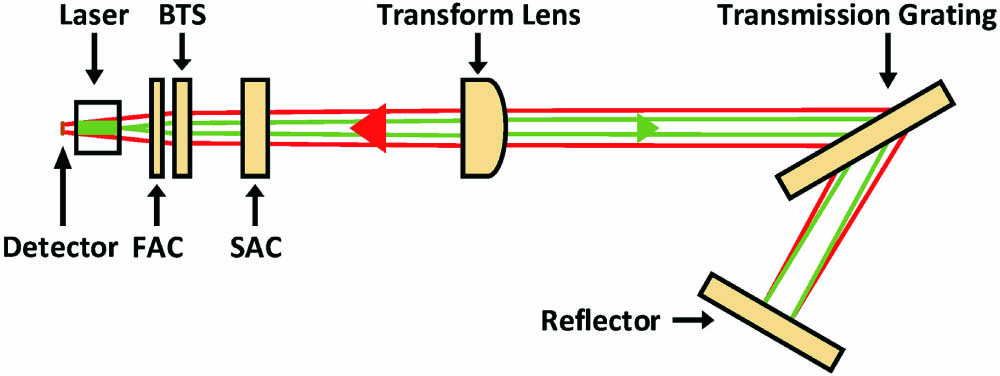
Set citation alerts for the article
Please enter your email address