Abstract
A ion-doped single crystal of high quality is grown successfully by an improved flux Bridgman method under the conditions of taking the chemical raw composition of in the molar ratio of , where the KF is shown to be an effective assistant flux. The x ray diffraction, absorption spectra, excitation spectra, and emission spectra of the -doped single crystal are measured to investigate the phase and optical properties of the single crystals. The absorption spectrum of the shows a strong band that peaks at the wavelength of 300 nm. The emission spectrum of the emits an intense ultraviolet (UV) band at the wavelength of 332 nm under the excitation of 300 nm light. Two separated luminous bands of 330 and 350 nm, which correspond to the transitions and In recent decades, the development of ultraviolet (UV) solid-state lasers has attracted great interest for their advantages of stable physical and chemical properties, narrow fluorspar spectral lines, strong absorption band, and high fluorescence quantum efficiency. UV lasers based on solid-state materials have many practical applications, such as medical procedures, semiconductor processing, optical communications and remote sensing, and so on. Although various kinds of lasers and tunable color center lasers have been investigated as UV sources, their applications are still restricted because many of them only operate at low temperatures and some of their materials deteriorate[1,2].
Rare earth ion-doped solid-state materials are mainly considered for devices due to the abundant energy levels of rare earth ions and the super properties of some of their matrixes. The ion is one of the most important active ions applied to UV lasers because of the transition around 325 nm[3]. The optical spectra and UV lasers based on ion-doped single crystals, glasses, and glass ceramics have already been studied[3–7].
Besides the rare earth ion dopant, the host material also plays an important role in the doping concentration, luminescence efficiency, thermal stability, etc. In the reported ion-doped materials, fluoride single crystals such as were mostly mentioned due to their low phonon energy, good chemical and thermal stability, high transparency at UV wavelengths, and high luminous efficiency[3,8].
Sign up for Chinese Optics Letters TOC. Get the latest issue of Chinese Optics Letters delivered right to you!Sign up now
crystals, such as the cubic and the hexagonal , have been widely studied for their application as excellent host for up-conversion[2] because of their low maximum phonon energy, which is about . More recently, bulk single crystals doped with [9], [10], and [11] were successfully grown in our laboratory by an improved flux Bridgman method. The transmittance in the UV wavelength and the Raman spectra of an single crystal have already recorded[9–11]. Compared with single crystals, the has a lower-value maximum phonon energy, about , and a higher transparency in the UV range[2,8]. The excellent properties of single crystals in thermal, mechanical, chemical, and optical behaviors are beneficial for its application in UV lasers to obtain a high luminous efficiency and high stability. However, there have been few reports for ion-doped bulk single crystals for UV lasers because of the difficulty of the crystal growth. In this Letter, the was incorporated in an single crystal. The concentration of in the single crystal was measured, and the optical performance of the obtained single crystal was explored to get a better-quality UV emission.
The doped single crystal was grown by a vertical Bridgman method using KF as the flux in a system. The raw materials were commercially available powders of high purity NaF (99.99%), KF (99.99%), (99.99%), and (99.99%). The moisture and the some oxygen impurities in the fluoride powders could be removed completely using the high-temperature hydrofluorinating method, by which the powders were sintered with anhydrous HF at 750°C for 8 h. The molar ratio of the powders was . Then, the mixture of those materials was ground about an hour in a mortar. The growth orientation was along the -axis by using an oriented seed crystal. The temperature gradient cross-solid-liquid interface was 70–90°C/cm; the growth process was carried on by lowering the crucible at a rate of . The detailed process of crystal growth is described elsewhere[9,10]. The obtained crystal shows high transparency. However, a white region of several centimeters in length which is main KF, , and some deviating components excluded from the melt exists at the top of the boule, corresponding to the final portion of the melt to freeze. The as-grown crystal was cut into pieces and well polished. The inset of Fig. 1 shows a polished piece of crystal. An fxamination using a polarizing microscope showed that the single crystal part is free from macroscopic defects.
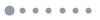
Figure 1.XRD pattern of (a) crystal and (b) literature data of the corresponding bulk materials with crystal structure for (JCPDS 77-2042).
The concentration in the single crystal was measured by an inductive coupled plasma (ICP) atomic emission spectrometer (PerkinElmer Inc., Optima 3000) and was about . The phase composition of the as-obtained crystals was recorded by x ray diffraction (XRD) using an XD-98X diffractometer (XD-3, Beijing). The scan was performed in the range from 10°–90° with a scanning step size of 0.02° and a 6 s swept time. A Cary 5000 UV/VIS/NIR spectrophotometer (Agilent Co., America) recorded the absorption spectra ranging from 200 to 600 nm. The optical properties of the single crystal doped with ions were characterized by excitation spectra and photoluminescence spectra measured using an F-4500 spectrophotometer (Hitachi high-technologies Co., Tokyo, Japan). All the above measurements were carried out at room temperature, and the samples were under the same conditions to get comparable results.
The phase compositions of the as-prepared sample were analyzed by powder XRD. Figure 1(a) shows the XRD patterns of the sample. Figure 1(b) shows the XRD patterns of card JCPD 77-2042[10,11]. It confirms that the transparent crystal obtained is an single crystal and that KF was excluded from the final part of the boule. Notably, compared with card JCPD 77-2042, the peak positions and intensity pattern matched well. It also confirms that the current ion doping level and introduction of ions do not bring any meaningful changes to the single crystal structure. As is well known, an single crystal has a fluorite structure (), in which and ions are randomly distributed in the cation sites, which are situated in the cubic center, and its lattice constants could be calculated from the XRD pattern data as the following lattice parameters[12]: for the lower part of the single crystal corresponding to the initial stage of crystal growth, and for the upper part of the single crystal corresponding to the final stage of crystal growth, which are close to the standard parameters as shown in Fig. 1 ().
The absorption spectra of -doped single crystals are shown in Fig. 2. It is obvious that the single crystals have only one intense absorption peak located at 300 nm. The absorption peak represents the absorption transition of ion in an single crystal from the ground state to the excitation state, viz, the electronic transition. In contrast, the single crystal doped with grown by the Czochralski method shows the absorption peak at 297 nm[3]. They are almost similar. Figures 3(a) and 3(b) display the excitation spectrum of the -doped single crystal by measuring the emission at 332 nm and the emission spectrum for the -doped single crystal under excitation at 300 nm wavelengths. It can be noted from Figs. 3(a) and 3(b) that there exists an excitation band at and an strong, sharp UV emission band at the wavelength of 332 nm.
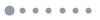
Figure 2.UV absorption spectra of the single crystal.
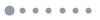
Figure 3.Excitation spectra of the single crystal (curve a) and the emission spectra of the single crystal (curve b).
It is known that the stable state of the rare-earth ion has a configuration and the excited state has a configuration[13–15]. The configuration is in the configuration and configuration. Thus, interference from the surrounding plasma electric field is small, and its energy level splitting obeys the spin orbit. The configuration splits into two levels with and for the spin-orbit (LS) coupling. The first excited state of ions is the configuration; because the configuration is more sensitive to the environment, the strong electro-acoustic coupling divides the configuration into five configurations at room temperature. Because the electronic transition is allowed, the wavelength of 332 nm belongs to the electric dipole transition of the electronic transition and it can be seen as consisting of two broadbands. All luminescence spectra can be fitted by two Gaussian functions corresponding to the luminescence transitions from the lowest state to the and levels of the state[3]. Figure 4 shows fitted results of the luminescence of by two Gaussian functions corresponding to the emission spectrum of the excitation wavelength of 300 nm. The fitted results indicate that the centers of the and luminescence transitions are at 330 and 350 nm, respectively. The splitting energy of about between the and levels can be reflected from the two emission peaks. Table 1 shows the fitted results of the luminescence spectra of the single crystal.
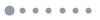
Figure 4.Fitted results of luminescence spectra of ion-doped single crystal excited by 300 nm wavelength. The inset is the energy level of a ion.
| Peak wavelength |
---|
Excitation source | Peak: 5d→F5/22 | Peak: 5d→F7/22 |
---|
300 nm | 330 nm | 350 nm |
30303 cm−1 | 28571 cm−1 |
Table 1. Fitted Results of Emission Spectra of the Ce3+:α-NaYF4 Single Crystal Excited by 300 nm Wavelength
In conclusion, the ion can be incorporated into an single crystal by a modified flux Bridgman method. When excited by the wavelength of 300 nm light, the emission spectra of the single crystals doped with ions can emit an intense beam of UV at the wavelength of 332 nm, which is composed of 330 and 335 nm broadbands and which is due to the electric-dipole transition of the electronic transition. The strong emission band at UV wavelengths and the super chemical-physical properties indicate that the ion-doped single crystal has potential applications in UV lasers.
References
[1] Y. L. Yin, C. M. Xu, L. C. Xu. Liaoning Chem. Ind., 38, 46(2009).
[2] J. He, G. Ren, Y. Mao, L. Xiang. Opt. Commun., 323, 174(2014).
[3] C. H. Huang, Q. L. Zhang, D. Zhou, Y. Li, S. T. Yin, C. S. Shi. J. Rare Earth, 32, 295(2003).
[4] M. Nikl, K. Nitsch, E. Mihokova, N. Solovieva, J. A. Mares, P. Fabeni, G. P. Pazzi, M. Martini, A. Vedda, S. Baccaro. Appl. Phys. Lett., 77, 2159(2000).
[5] Q. Wang, S. Ouyang, W. Zhang, B. Yang, Y. Zhang, H. Xia. J. Rare Earths, 33, 13(2015).
[6] Y. G. Choi, K. H. Kim, S. H. Park, J. Heo. J. Appl. Phys., 88, 3832(2000).
[7] A. Vedda, N. Chiodini, D. Di Martino, M. Fasoli, S. Keffer, A. Lauria, M. Martini, F. Moretti, G. Spinolo, M. Nikl, N. Solovieva, G. Brambilla. Appl. Phys. Lett., 85, 6356(2004).
[8] F. Okada, S. Togawa, K. Ohta, S. Koda. J. Appl. Phys., 75, 49(1994).
[9] J. Zhang, H. Xia, Y. Jiang, S. Yang, H. Jiang, B. Chen. IEEE J. Quantum Electron., 51, 1(2015).
[10] J. Zhang, H. Xia, Y. Jiang, S. Yang, H. Jiang, B. Chen. J. Mater. Sci. Technol., 31, 1232(2015).
[11] S. Yang, H. Xia, Y. Jiang, J. Zhang, Y. Shi, X. Gu, J. Zhang, Y. Zhang, H. Jiang, B. Chen. J. Alloys Compd., 643, 1(2015).
[12] Y. Jiang, H. Xia, S. Yang, J. Zhang, D. Jiang, C. Wang, Z. Feng, J. Zhang, X. Gu, J. Zhang, H. Jiang, B. Chen. Chin. Opt. Lett., 13, 071601(2015).
[13] G. Blasse, A. Bril. J. Chem. Phys., 47, 5139(1967).
[14] Z. L. Wu, Y. F. Ruan, Y. F. Wang, S. Wang, H. S. Tong. J. Synth. Cryst., 41, 006(2012).
[15] X. H. Ceng, G. J. Zhao, L. H. Zhang, Y. Hang, H. J. Li, J. Xu. Acta Phys. Sin., 54, 612(2005).