Alexandr S. Korsakov, Alexandr E. Lvov, Dmitry S. Vrublevsky, and Liya V. Zhukova*
Abstract
For the development of mid-infrared fiber-optical elements, one needs light-stable, flexible materials that are transparent within this spectral band. Solid solutions of silver and monadic thallium halides prove to be the most suitable crystalline media for these needs. We study the light stability variation of high-purity () and () crystals by measuring the optical transmission change as functions of the composition and UV exposure time. The former is executed in a broad spectral range from , within which we choose three wavelengths to trace the transmission change. For thallium-monoiodide-containing samples, an effect is observed that we assumed to be translucence.Currently, the demand for quality special features of polycrystalline infrared (IR) fibers is getting higher. These features can be achieved by the creation of controllable defects in the crystals, which the IR fibers are further extruded from Refs. [1–5].
The search for, and manufacture of, real, or defective, crystals possessing various structure-sensitive properties, such as optical, luminescent, mechanical, magnetic, photoelectric, etc., are related to their composition and degree of defectiveness. The very term “defect” is referred to as a structural element of the crystal that defines the properties of the crystalline array. In their monograph, the authors[6] attribute interstitial and substitution solid solutions exactly to defective crystals, where the major chemical principles of crystals with defects are stated.
Crystalline media form a new class of materials that may be efficiently used in IR fiber optics. Recently, the crystalline fibers for the 2.0–25.0 μm range have been being reported on repeatedly[3,5,7]. They are usually manufactured by extrusion from AgCl-AgBr solid solution crystals, representing the only polycrystalline, non-toxic, and non-hygroscopic IR fibers for the needs of laser, endoscopic, and diagnostic medicine. However, such fibers have a drawback: they are more light sensitive (although they turned out to be more stable over a wide wavelength range) than individual AgCl and AgBr crystals[8]. Due to this imperfection, a search for some new compositions and their development is needed, and in this regard, silver and monadic thallium halide solid solutions seem suitable.
Sign up for Chinese Optics Letters TOC. Get the latest issue of Chinese Optics Letters delivered right to you!Sign up now
Thermodynamic research on phase crystal-melt equilibrium diagrams of heterogeneous AgBr-TlI system allowed us to estimate the region where the stable substitution solid solutions of the NaCl type exist at room temperature up to 14 mol% of TlI in AgBr-TlI[7]. By using certain technological approaches and equipment to synthesize raw materials and to grow crystals from them[9], and by relying upon the phase diagram, we obtain () solid solution crystals for further single and multimode IR fiber manufacturing. Crystals proved to be more light stable and to have a wider transparency window in the mid-IR compared with AgCl-AgBr crystals. Their cationic impurity content is less than weight % for Fe, Si, Pb, Sn, Zn, and In and for Mg, Al, Ni, Cr, and Mn.
The light stability, or photostability, investigation was performed by Fourier transform IR spectrometry (Shimadzu “IR-Prestige-21” with KBr divisor, DLaTGS detector, within the range and with a resolution). The transmission specrta were recorded before the samples were exposed to radiation, which was to be used as a background. Therefore, we collected the data on the relative decrease of the transmission intensity due to decomposition, followed by colloid formation[10]. To expose each of our plate-shaped samples, we put it in a special holder (Fig. 1), where it was irradiated by a broadband UV lamp with an intensity peak at 220–240 nm.
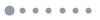
Figure 1.Setup for irradiation: 1: UV lamp, 2: protective sleeve, 3: exposed flat sample, and 4: plate holder.
It is seen from Fig. 2, where one of the samples is presented, that at the exposure point, a material modification took place, resulting in a thin, opaque film formation on the surface.
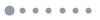
Figure 2.Pictures of : (a) before and after exposure to the UV lamp, (b) 500-fold magnified border between exposed and unexposed parts, and (c) 1000-fold magnification of the exposed area.
While for the AgCl-AgBr system this film was gray, which we attributed to the liberation of silver particles according to the mechanism described by the authors in Ref. [11], the surface film color for AgBr-TlI system appeared to be orange, even rubiginous. Moreover, this film tended to become more pronounced when increasing the thallium monoiodide content.
Figure 3 provides an example of the transmission spectrum right after irradiation, while the data on the transmission intensity reduction is depicted in Figs. 4 and 5. It is seen from the plots that the optical loss increase is inversely proportional to the wavelength. In the AgCl-AgBr system, the optical losses drop significantly in a wide spectral range when increasing the AgBr content, with the surface film color becoming dimmer. In contrast, when increasing the TlI content in the AgBr-TlI system, optical losses rise over the most parts of the spectrum, with the surface film color turning more vivid (Fig. 2(a)). However, within a smaller range of longer wavelengths, a transmission increase is observed, which we refer to as translucence. We assume that such an effect is similar to optical material translucence: the application of a single-layered or textured antireflective coating with a lower refractive index. The closer the refractive index is to the square root of that of the treated material, the stronger this effect becomes. The final single-layer thickness should amount to or be a multiple of a quarter of a wavelength[12]. Since we surveyed the translucence effect here and observed thin film formation (around 2 μm, see Figs. 2(b) and 2(c)), we assume its refractive index to be lower than the initial one, as said above. As seen from Figs. 2(b) and 2(c), and according to Ref. [10], this sparse surface film is made of metal particles, which had left the cation sublattice of the irradiated solid solution; these particles cause the losses at short wavelengths (and then at longer ones when aggregated) to rise. We do not exclude that the translucence effect takes place in the AgCl-AgBr system as well, but it never manifested itself. As for the AgBr-TlI system, this effect may be observed distinctly. The detailed investigation of this phenomenon will be the topic of our future research.
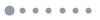
Figure 3.Relative transmission variation depending on the exposure time for .
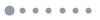
Figure 4.Optical losses versus UV exposure time of the AgCl-AgBr and AgBr-TlI plates at three different wavelengths.
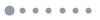
Figure 5.Relative change of transmission intensity in terms of optical losses as a function of the specific compositions of the AgCl-AgBr and AgBr-TlI solid solutions.
Thus, we investigated the light stability of AgCl-AgBr and AgBr-TlI systems of various substitution agent content and determined the optical loss dependence on the composition and time of exposure to UV rays.
The experiment was carried out over a wide spectral range from 6500 to , within which three distinct wavelengths, 2, 4.8, 10.6 μm, were chosen to keep track of the optical transmission variation. According to this data, when increasing the AgBr content in AgCl-AgBr, at up to almost 40 mol% at shorter wavelengths or up to around 14 mol% at longer ones, loss reduction takes place, followed by reaching a relatively constant loss value. Regarding the AgBr-TlI system, after increasing the TlI fraction, the rise of the optical losses in the short wavelength range is observed, while at longer wavelengths, one can see a small increase first, but then translucence takes place already from 2 mol% of TlI, reaching about 4% for 5 mol%. This effect may be accounted for as the formation of a surface antireflective layer with a lower refractive index. This layer may be considered as a dispersion system, probably formed by a sparse metal/metal oxide film and ambient air. Heavier ions, namely and , in the bulk allow for the spectral range widening towards longer wavelengths. However, this advantage is graded by the higher optical density and consequently, the increased Fresnel reflection at the material-air interface. The translucence effect we discovered probably would lower this reflection, thereby increasing the effectiveness of transmission through the optical element.
References
[1] L. Zhukova, A. Korsakov, D. Vrublevsky. New Infrared Materials: Crystals and Fibers, 280(2014).
[2] L. V. Zhukova, A. S. Korsakov, D. S. Vrublevsky, E. V. Zharikov, V. S. Korsakov. Nonferrous Met., 1, 69(2010).
[3] E. Rave, P. Ephrat, M. Goldberg, E. Kedmi, A. Katzir. Appl. Opt., 43, 2236(2004).
[4] O. Wallner, V. G. Artjuschenko, R. Flatscher. Proc. SPIE, 5491, 636(2004).
[5] S. Shalem, A. Tsun, E. Rave, A. Millo, L. Nagli, A. Katzir. Appl. Phys. Lett., 91103, 87(2005).
[6] P. V. Kovtunenko. Physical Chemistry of Solids, the Crystals with Defects(1993).
[7] A. Korsakov, L. Zhukova, D. Salimgareev, V. Zhukov. Chin. Opt. Lett., 13, 090602(2015).
[8] A. Korsakov, L. Zhukova. Crystals for the Infrared Fiber Optics. Physical and Chemical Grounds for Silver and Thallium (I) Halide Solid Solution Synthesis for IR Fiber Optics(2011).
[9] A. Korsakov, L. Zhukova, E. Korsakova, E. Zharikov. J. Cryst. Growth, 386, 94(2014).
[10] L. G. Grigoryeva, D. K. Millers, E. A. Kotomin, R. I. Eglitis, A. A. Lehrman. J. Phys. D.: Appl. Phys., 29, 578(1996).
[11] I. Pelant, J. Valenta. Luminescence Spectroscopy of Semiconductors(2012).
[12] M. Born, E. Wolf. Principles of Optics(1980).