Author Affiliations
1State Key Laboratory of Information Photonics and Optical Communications, School of Science, P. O. Box 91, Beijing University of Posts and Telecommunications, Beijing 100876, China2Beijing National Laboratory for Condensed Matter Physics, Institute of Physics, Chinese Academy of Sciences, Beijing 100190, Chinashow less
Abstract
Tungsten disulfide (WS2) is a type of anisotropic-layered compound and has broadband saturable absorption features as saturable absorbers (SAs). With WS2-based SAs, dark solitons in erbium-doped fiber (EDF) lasers are first obtained. For the generated dark solitons, the center wavelength is measured to be 1530 nm, and the repetition rateis about 116.5 MHz. A series of optical spectra is exhibited. The electrical signal-to-noise ratio is better than 94 dB. Results in this paper demonstrate that WS2-based SAs are the promising SAs for generating dark solitons in EDF lasers.1. INTRODUCTION
Ultrafast fiber lasers can be used in material processing and optical communications [1–4]. They can be divided into two types: actively mode-locked fiber lasers and passively mode-locked fiber lasers. Compared with actively mode-locked fiber lasers, passively ones have the advantages of compactness, simplicity, and flexibility [5]. Due to the fast amplitude modulation, two techniques, i.e., semiconductor saturable absorber mirror (SESAM) and nonlinear polarization rotation (NPR), have been extensively studied [6,7]. However, the SESAM technique requires complicated manufacturing technology and packaging process and has narrow operation bandwidth [6]. The NPR technique suffers from bulky construction and environmental sensitivity [7]. To seek for new and high-performance saturable absorbers (SAs), 2D nano-materials as saturable absorption materials have been used to fabricate SAs for passively mode-locked fiber lasers [8–10].
Among those SAs, carbon-based SAs, such as carbon nanotubes (CNTs) and graphene, which have the advantages of ultrafast recovery time, easy fabrication, and low cost, have been investigated to generate ultrashort pulses [11–14]. However, CNTs SAs are wavelength dependent on their diameters, and can lead to strong nonsaturable losses [11,13]. For graphene, the absorption is weak, which would decrease the SAs’ modulation ability [15]. Thus, new SAs based on other 2D nano-materials have been developed [16–28]. The new 2D nano-materials for the SAs mainly include topological insulators (TIs) [16–19], transition mental dichalcogenides (TMDs) [20–26], and black phosphorus [27,28]. For TIs, the optical absorbance decreased when the incident laser intensity increased, and TI SAs were saturated above a certain threshold [29,30]. The tungsten disulfide ()-based SA can overcome those disadvantages. Therefore, the -based SA has been investigated as a new substitution for other SAs, and the fiber lasers with -based SAs have been constructed [31–35].
On the other hand, compared with bright solitons, dark ones are less sensitive to fiber loss and more stable in the presence of noise [36–38]. Therefore, dark solitons have been experimentally generated in fiber lasers at various center wavelengths and have been recently investigated [39–54]. However, so far dark solitons in EDF lasers with -based SAs have not been reported.
Sign up for Photonics Research TOC. Get the latest issue of Photonics Research delivered right to you!Sign up now
Here, the first generation of dark solitons in EDF lasers with -based SAs will be studied. Combining -based SAs and the NPR technique, dark solitons in the EDF lasers will be observed. A series of optical spectra of them will be first presented. In addition, the signal-to-noise ratio (SNR) will be measured.
2. SETUP OF PASSIVELY MODE-LOCKED EDF LASERS WITH WS2-BASED SAS
The setup of our fiber laser is presented in Fig. 1. The length of erbium-doped fiber (EDF) (Liekki 110-4/125) is 260 mm. Introduction of the working principle for Fig. 1 can be found in [47]. The -based SA, which is inserted between the EDF and wavelength-division multiplexer (WDM), is the same as used and described in [5]. The total cavity length is about 1.9 m. In the process of testing related parameters, we have added the half-wave plate (HWP) and polarization beam splitter (PBS) to make the output pulse into two parts: photodetector (PD)1 for monitoring, and PD2 for parameter measurement. By rotating the HWP between two PBS, we can adjust the incident power into PD1 and PD2, respectively.
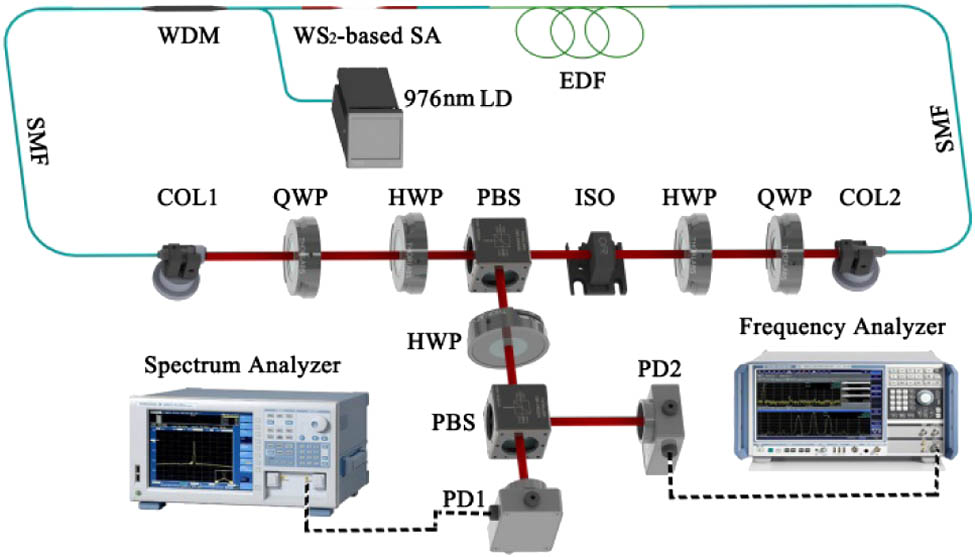
Figure 1.Schematic of the mode-locked EDF laser. SMF, single mode fiber; COL, collimator; QWP, quarter-wave plate; ISO, polarization-dependent isolator.
3. EXPERIMENTAL RESULTS AND DISCUSSION
By rotating HWPs and QWPs in Fig. 1, we can obtain the different optical spectra of dark solitons as shown in Fig. 2. The fiber laser is mode-locking through -based SAs and NPR technique, and the mode-locking threshold is about . Compared with NPR fiber lasers, the mode-locking threshold is low due to -based SAs. The center wavelength of the optical spectrum is 1530 nm. If we maintain the pump power above the threshold of mode-locking and only adjust HWPs and QWPs, the different optical spectra of dark solitons can be obtained. Generally, when optical pulses propagate through HWPs and QWPs, their polarization states could have a change relying on the retardation between the slow and fast axes, and the intracavity power of fiber lasers could be adjusted.
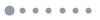
Figure 2.Different optical spectra of dark solitons are obtained with the different polarization states when we rotate HWPs and QWPs in the fiber laser. (a) The optical spectrum is smooth with the 4.8 nm spectral width at 1530 nm. (b) The optical spectrum is a bit rough with the 4.1 nm spectral width. (c) and (d) both have the 0.16 nm spectral width.
For Fig. 2(a), the 3 dB spectral width is 4.8 nm, which has a large intracavity power in fiber lasers. The optical spectrum is relatively smooth. Rotating HWPs and QWPs to decrease the intracavity power, the relatively narrow optical spectrum is presented in Fig. 2(b), and the optical spectrum is a bit rough with the 4.1 nm spectral width. Because the intracavity power is high, high-order solitons are formed. Figures 2(a) and 2(b) show their optical spectra. If we decrease the intracavity power, the optical spectra of fundamental solitons appear as in Figs. 2(c) and 2(d). We present the corresponding pulse sequences on the time scales of 10 ns/div in Fig. 3. We can see the splitting of high-order solitons in Figs. 3(a) and 3(b) due to the high intracavity power.
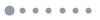
Figure 3.Due to the different intracavity power, the different pulse sequences corresponding to Fig. 2 are presented. The oscilloscope trace of dark solitons is in the time scales of 10 ns/div.
Dark solitons emit from the intracavity PBS with the repetition rate of 116.5 MHz in Fig. 4(a), which is measured by a radio frequency analyzer (ROHDE & SCHWARZ FSW26). With 10 kHz resolution bandwidth (RBW), the SNR is better than 94 dB, which demonstrates that the mode-locking state is quite stable. The reason for the stability is that -based SAs can acted as the nonlinear filter for the pulse amplitude and avoid excessive intracavity nonlinear. Integrated from 1 MHz down to 10 Hz, the timing jitter is about 1 ps. Compared with [47] (the SNR is about 63 dB, and the timing jitter is about 10 ps), this fiber laser has better performance than the TI fiber laser. During this measurement, our fiber laser is no encapsulation. The lock circuit is also not used. That is to say, incorporating the -based SA in fiber lasers can enhance the environmental stability.
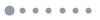
Figure 4.(a) Radio frequency (RF) spectrum of dark solitons. (b) Phase noise measurement at 116.5 MHz, and the timing jitter is about 1 ps.
4. CONCLUSION
In conclusion, we have demonstrated dark solitons with -based SAs in the fiber laser and carried out the hybrid mode locking. The repetition rate of dark solitons has been 116.5 MHz at 1530 nm. Besides, due to -based SAs, the mode-locking threshold has been about 60 mW, which is relatively low compared with NPR fiber lasers. With the same pump power, the different optical spectra of dark solitons have been obtained by properly rotating HWPs and QWPs. The corresponding pulse sequences have been listed. The timing jitter and SNR have been measured to be 1 ps and 94 dB, respectively. Results indicate that the optical spectra of dark solitons can be diverse, and the mode-locked EDF laser can easily generate stable dark solitons with -based SAs.
ACKNOWLEDGMENT
Acknowledgment. We express our sincere thanks to the editors and referees for their valuable comments. We also express our sincere thanks to Peiguang Yan for providing us the WS2-based SAs. This work has been supported by the National Key Basic Research Program of China (grant nos. 2012CB821304, 2013CB922401, and 2013CB922402), by the National Natural Science Foundation of China (NSFC) (grant nos. 61205064, 61378040, and 11078022), by the National Key Technology R&D Program of the Ministry of Science and Technology under grant no. 2012BAC23B03, and by the Fund of State Key Laboratory of Information Photonics and Optical Communications (Beijing University of Posts and Telecommunications, grant no. 600100161).
References
[1] U. Keller. Recent developments in compact ultrafast lasers. Nature, 424, 831-838(2003).
[2] B. Oktem, C. Ulgudur, F. O. Ilday. Soliton-similariton fibre laser. Nat. Photonics, 4, 307-311(2010).
[3] X. M. Liu, Y. D. Cui, D. D. Han, X. K. Yao, Z. P. Sun. Distributed ultrafast fibre laser. Sci. Rep., 5, 9101(2015).
[4] P. G. Yan, A. J. Liu, Y. S. Chen, J. Z. Wang, S. C. Ruan, H. Chen, J. F. Ding. Passively mode-locked fiber laser by a cell-type WS2 nanosheets saturable absorber. Sci. Rep., 5, 12587(2015).
[5] P. G. Yan, A. J. Liu, Y. S. Chen, H. Chen, S. C. Ruan, C. Y. Guo, S. F. Chen, I. L. Li, H. P. Yang, J. G. Hu, G. Z. Cao. Microfiber-based WS2-film saturable absorber for ultra-fast photonics. Opt. Mater. Express, 5, 479-489(2015).
[6] U. Keller, K. J. Weingarten, F. X. Kartner, D. Kopf, B. Braun, I. D. Jung, R. Fluck, C. Honninger, N. Matuschek, J. Aus der Au. Semiconductor saturable absorber mirrors (SESAM’s) for femtosecond to nanosecond pulse generation in solid state lasers. IEEE J. Sel. Top. Quantum Electron., 2, 435-453(1996).
[7] L. Nelson, D. Jones, K. Tamura, H. Haus, E. Ippen. Ultra short pulse fiber ring lasers. Appl. Phys. B, 65, 277-294(1997).
[8] P. G. Yan, R. Y. Lin, H. Chen, H. Zhang, A. J. Liu, H. P. Yang, S. C. Ruan. Topological insulator solution filled in photonic crystal fiber for passive mode-locked fiber laser. IEEE Photon. Technol. Lett., 27, 264-267(2015).
[9] P. Grelu, N. Akhmediev. Dissipative solitons for mode-locked lasers. Nat. Photonics, 6, 84-92(2012).
[10] L. Li, S. Z. Jiang, Y. G. Wang, X. Wang, L. N. Duan, D. Mao, Z. Li, B. Y. Man, J. H. Si. WS2/fluorine mica (FM) saturable absorbers for all-normal-dispersion mode-locked fiber laser. Opt. Express, 23, 28698-28706(2015).
[11] S. Yamashita, Y. Inoue, S. Maruyama, Y. Murakami, H. Yaguchi, M. Jablonski, S. Set. Saturable absorbers incorporating carbon nanotubes directly synthesized onto substrates and fibers and their application to mode locked fiber lasers. Opt. Lett., 29, 1581-1583(2004).
[12] F. Wang, A. G. Rozhin, V. Scardaci, Z. Sun, F. Hennrich, I. H. White, W. I. Milne, A. C. Ferrari. Wideband-tuneable, nanotube mode-locked, fibre laser. Nat. Nanotechnol., 3, 738-742(2008).
[13] T. Hasan, Z. Sun, F. Wang, F. Bonaccorso, P. H. Tan, A. G. Rozhin, A. C. Ferrari. Nanotube-polymer composites for ultrafast photonics. Adv. Mater., 21, 3874-3899(2009).
[14] X. M. Liu, D. D. Han, Z. P. Sun, C. Zeng, H. Lu, D. Mao, Y. D. Cui, F. Q. Wang. Versatile multi-wavelength ultrafast fiber laser mode-locked by carbon nanotubes. Sci. Rep., 3, 2718(2013).
[15] Z. C. Luo, M. Liu, Z. N. Guo, X. F. Jiang, A. P. Luo, C. J. Zhao, X. F. Yu, W. C. Xu, H. Zhang. Microfiber-based few-layer black phosphorus saturable absorber for ultra-fast fiber laser. Opt. Express, 23, 20030-20039(2015).
[16] L. P. Sun, Z. Q. Lin, J. Peng, J. Weng, Y. Z. Huang, Z. Q. Luo. Preparation of few-layer bismuth selenide by liquid-phase-exfoliation and its optical absorption properties. Sci. Rep., 4, 4794(2014).
[17] P. G. Yan, R. Y. Lin, S. C. Ruan, A. J. Liu, H. Chen, Y. Q. Zheng, S. F. Chen, C. Y. Guo, J. G. Hu. A practical topological insulator saturable absorber for mode-locked fiber laser. Sci. Rep., 5, 8690(2015).
[18] S. B. Lu, C. J. Zhao, Y. H. Zou, S. Q. Chen, Y. Chen, Y. Li, H. Zhang, S. C. Wen, D. Y. Tang. Third order nonlinear optical property of Bi2Se3. Opt. Express, 21, 2072-2082(2013).
[19] Z. C. Luo, M. Liu, H. Liu, X. W. Zheng, A. P. Luo, C. J. Zhao, H. Zhang, S. C. Wen, W. C. Xu. 2 GHz passively harmonic mode-locked fiber laser by a microfiber-based topological insulator saturable absorber. Opt. Lett., 38, 5212-5215(2013).
[20] H. Zhang, S. B. Lu, J. Zheng, J. Du, S. C. Wen, D. Y. Tang, K. P. Loh. Molybdenum disulfide (MoS2) as a broadband saturable absorber for ultra-fast photonics. Opt. Express, 22, 7249-7260(2014).
[21] R. I. Woodward, E. J. R. Kelleher, R. C. T. Howe, G. Hu, F. Torrisi, T. Hasan, S. V. Popov, J. R. Taylor. Tunable Q-switched fiber laser based on saturable edge-state absorption in few-layer molybdenum disulfide (MoS2). Opt. Express, 22, 31113-31122(2014).
[22] M. Liu, X. W. Zheng, Y. L. Qi, H. Liu, A. P. Luo, Z. C. Luo, W. C. Xu, C. J. Zhao, H. Zhang. Microfiber-based few-layer MoS2 saturable absorber for 2.5 GHz passively harmonic mode-locked fiber laser. Opt. Express, 22, 22841-22846(2014).
[23] R. Khazaeizhad, S. H. Kassani, H. Jeong, D. I. Yeom, K. Oh. Mode-locking of Er-doped fiber laser using a multilayer MoS2 thin film as a saturable absorber in both anomalous and normal dispersion regimes. Opt. Express, 22, 23732-23742(2014).
[24] Q. H. Wang, K. Kalantar-Zadeh, A. Kis, J. N. Coleman, M. S. Strano. Electronics and optoelectronics of two-dimensional transition metal dichalcogenides. Nat. Nanotechnol., 7, 699-712(2012).
[25] D. Mao, Y. Wang, C. Ma, L. Han, B. Jiang, X. Gan, S. Hua, W. Zhang, T. Mei, J. Zhao. WS2 mode-locked ultrafast fiber laser. Sci. Rep., 5, 7965(2015).
[26] R. I. Woodward, R. C. T. Howe, T. H. Runcorn, G. Hu, F. Torrisi, E. J. R. Kelleher, T. Hasan. Wideband saturable absorption in few-layer molybdenum diselenide (MoSe2) for Q-switching Yb-, Er- and Tm-doped fiber lasers. Opt. Express, 23, 20051-20061(2015).
[27] J. Sotor, G. Sobon, W. Macherzynski, P. Paletko, K. M. Abramski. Black phosphorus saturable absorber for ultrashort pulse generation. Appl. Phys. Lett., 107, 051108(2015).
[28] Y. Chen, G. B. Jiang, S. Q. Chen, Z. N. Guo, X. F. Yu, C. J. Zhao, H. Zhang, Q. L. Bao, S. C. Wen, D. Y. Tang, D. Y. Fan. Mechanically exfoliated black phosphorus as a new saturable absorber for both Q-switching and mode-locking laser operation. Opt. Express, 23, 12823-12833(2015).
[29] C. Zhao, H. Zhang, X. Qi, Y. Chen, Z. Wang, S. C. Wen, D. Y. Tang. Ultra-short pulse generation by a topological insulator based saturable absorber. Appl. Phys. Lett., 101, 211106(2012).
[30] H. Liu, A. P. Luo, F. Z. Wang, R. Tang, M. Liu, Z. C. Luo, W. C. Xu, C. J. Zhao, H. Zhang. Femtosecond pulse erbium-doped fiber laser by a few-layer MoS2 saturable absorber. Opt. Lett., 39, 4591-4594(2014).
[31] K. G. Zhou, M. Zhao, M. J. Chang, Q. Wang, X. Z. Wu, Y. Song, H. L. Zhang. Size-dependent nonlinear optical properties of atomically thin transition metal dichalcogenide nanosheets. Small, 11, 694-701(2015).
[32] X. Zheng, Y. Zhang, R. Chen, X. Cheng, Z. Xu, T. Jiang. Z-scan measurement of the nonlinear refractive index of monolayer WS2. Opt. Express, 23, 15616-15623(2015).
[33] K. Wu, X. Zhang, J. Wang, X. Li, J. Chen. WS2 as a saturable absorber for ultrafast photonic applications of mode-locked and Q-switched lasers. Opt. Express, 23, 11453-11461(2015).
[34] S. H. Kassani, R. Khazaeinezhad, H. Jeong, T. Nazari, D. I. Yeom, K. Oh. All-fiber Er-doped Q-Switched laser based on Tungsten disulfide saturable absorber. Opt. Express, 5, 373-379(2015).
[35] M. Jung, J. Lee, J. Park, J. Koo, Y. M. Jhon, J. H. Lee. Mode-locked, 1.94-μm, all-fiberized laser using WS2-based evanescent field interaction. Opt. Express, 23, 19996-20006(2015).
[36] W. Zhao, E. Bourkoff. Generation, propagation, and amplification of dark solitons. J. Opt. Soc. Am. B, 9, 1134-1144(1992).
[37] C. Milián, D. V. Skryabin, A. Ferrando. Continuum generation by dark solitons. Opt. Lett., 34, 2096-2098(2009).
[38] H. H. Liu, K. K. Chow. Dark pulse generation in fiber lasers incorporating carbon nanotubes. Opt. Express, 22, 29708-29713(2014).
[39] X. L. Li, S. M. Zhang, Y. C. Meng, Y. P. Hao. Harmonic mode locking counterparts of dark pulse and dark-bright pulse pairs. Opt. Express, 21, 8409-8416(2013).
[40] Y. S. Kivshar, B. Luther-Davies. Dark optical solitons: physics and applications. Phys. Rep., 298, 81-197(1998).
[41] P. Emplit, J. P. Hamaide, R. Reynaud, C. Froehly, A. Barthelemy. Picosecond steps and dark pulses through nonlinear single mode fibers. Opt. Commun., 62, 374-379(1987).
[42] W. Zhao, E. Bourkoff. Propagation properties of dark solitons. Opt. Lett., 14, 703-705(1989).
[43] H. Zhang, D. Y. Tang, L. M. Zhao, R. J. Knize. Vector dark domain wall solitons in a fiber ring laser. Opt. Express, 18, 4428-4433(2010).
[44] D. Y. Tang, J. Guo, Y. F. Song, H. Zhang, L. M. Zhao, D. Y. Shen. Dark soliton fiber lasers. Opt. Express, 22, 19831-19837(2014).
[45] Y. Q. Ge, J. L. Luo, L. Li, X. X. Jin, D. Y. Tang, D. Y. Shen, S. M. Zhang, L. M. Zhao. Initial conditions for dark soliton generation in normal-dispersion fiber lasers. Appl. Opt., 54, 71-75(2015).
[46] G. D. Shao, Y. F. Song, J. Guo, L. M. Zhao, D. Y. Shen, D. Y. Tang. Induced dark solitary pulse in an anomalous dispersion cavity fiber laser. Opt. Express, 23, 28430(2015).
[47] W. J. Liu, L. H. Pang, H. N. Han, W. L. Tian, H. Chen, M. Lei, P. G. Yan, Z. Y. Wei. Generation of dark solitons in erbium-doped fiber lasers based Sb2Te3 saturable absorbers. Opt. Express, 23, 26023-26031(2015).
[48] H. Zhang, D. Y. Tang, L. M. Zhao, X. Wu. Dark pulse emission of a fiber laser. Phys. Rev. A, 80, 045803(2009).
[49] X. Wang, P. Zhou, X. Wang, H. Xiao, Z. Liu. 2 μm bright-dark pulses in Tm-doped fiber ring laser with net anomalous dispersion. Appl. Phys. Express, 7, 022704(2014).
[50] D. Y. Tang, L. Li, Y. F. Song, L. M. Zhao, H. Zhang, D. Y. Shen. Evidence of dark solitons in all-normal dispersion fiber lasers. Phys. Rev. A, 88, 013849(2013).
[51] D. J. Richardson, R. P. Chamberlin, L. Dong, D. N. Payne. Experimental demonstration of 100 GHz dark soliton generation and propagation using a dispersion decreasing fibre. Electron. Lett., 30, 1326-1327(1994).
[52] A. K. Atieh, P. Myslinske, J. Chrostowski, P. Galko. Generation of multigigahertz bright and dark soliton pulse trains. Opt. Commun., 133, 541-548(1997).
[53] T. Sylvestre, S. Coen, P. Emplit, M. Haelterman. Self-induced modulational instability laser revisited: normal dispersion and dark-pulse train generation. Opt. Lett., 27, 482-484(2002).
[54] Y. F. Song, J. Guo, L. M. Zhao, D. Y. Shen, D. Y. Tang. 280 GHz dark soliton fiber laser. Opt. Lett., 39, 3484-3487(2014).