Abstract
We extensively discuss 25 Gb/s per wavelength capacity in both IEEE and ITU-T standardization to support the increasing bandwidth requirement. In this Letter, we propose to use the optical dispersion compensation technique in an optical line terminal (OLT) combined with a bandwidth-limited electro-absorption modulated laser in an optical network unit to achieve 25 Gb/s capacity for the upstream link. We evaluate the positive and negative dispersion tolerances of 25 Gb/s electrical duo-binary (EDB) and pulse-amplitude modulation (PAM-4) signals. We achieve 39.5 and 31 dB upstream loss budgets for the 25 Gb/s EDB and PAM-4 signals by using and optical dispersion compensation in OLT, respectively, both supporting 0–40 km differential reach.As the bandwidth demand of emerging high-quality multimedia applications keeps increasing, it is essential to developing next-generation passive optical networks beyond 10 Gb/s to satisfy the end user’s bandwidth demand in the near future. For next-generation access technologies, 25 Gb/s per wavelength has been considered to support 100 Gb/s capacity for both ITU-T and IEEE. Various solutions have been proposed to realize 100 Gb/s passive optical network (PON) systems by using high-order modulation formats, such as quaternary level pulse-amplitude modulation (PAM-4)[1–3], electrical duo-binary (EDB)[4–6], and optical duo-binary (ODB)[7]. Non-return-to-zero (NRZ) has also been proposed as the downstream modulation format option[8,9], but after the bandwidth-limited receiver in the optical network unit (ONU), it is still in the EDB format, and only the detection algorithms are different. Therefore, we still consider this NRZ format as a variant of EDB. The ODB modulation scheme is beneficial to the fiber transmission as a result of the chromatic dispersion (CD) tolerance characteristic. But a high-bandwidth Mach−Zehnder modulator and a bandwidth receiver with an equal data rate are required, which increase the expenditure cost and limits its use in the optical line terminal (OLT). Until now, the modulation format options have not been finalized yet, but most of the research has been focused on the downstream direction. For a 25 Gb/s data rate in the upstream direction, ODB should not be a choice due to its high cost in the ONU; therefore, EDB or PAM-4 will be the options based on 10 G-class devices. Since the C-band is also a choice for the upstream wavelength, dispersion compensation is required to support a 0–40 km differential reach. Burst-mode electrical dispersion compensation has been proposed to compensate for the 0–40 km differential reach[10], and we have proposed to use optical dispersion compensation (ODC) in the OLT to compensate for the 0–100 km differential reach[11] for upstream 10 Gb/s directly modulated signals. However, there have been no demonstrations on the dispersion compensation to support a 0–40 km differential reach, which means supporting all the users distributed within 40 km for 25 Gb/s upstream signals. In this Letter[2], the reach is doubled from 20 to 40 km by using a dispersion compensation fiber (DCF) at the OLT side for the downstream. But it is not the optimal dispersion compensation value for the users of 0–40 km.
In this Letter, we employ ODC in an OLT to compensate for the CD of a 0–40 km differential reach of both 25 Gb/s EDB and PAM-4 signals. We evaluate the dispersion tolerance of the 25 Gb/s EDB and PAM-4 signals on both the positive and negative dispersion values in order to find the optimal dispersion value in the OLT and compare the upstream loss budget for the two modulation formats at its ODC value. Finally, we achieved 39.5 and 31 dB loss budgets for the EDB and PAM-4 formats at and dispersions at the OLT, respectively. Considering the fact that the burst-mode duobinary receiver is already available[2] and the ODC can support multiple channels, we conclude an electro-modulation laser (EML)-based EDB format with an ODC in the OLT would be a good candidate for the upstream in a symmetrical 100 G-PON in the C-band. Note that the 39.5 dB upstream loss budget is also the record value for 25 Gb/s time-division-multiplexed PON (TDM-PON).
As for the downstream solution of 100 G-PON, we have demonstrated the first field trial of a real-time 100 Gb/s TWDM-PON system with downstream and upstream transmissions using 10 G-class DMLs and APD/PIN receivers with a power budget of 33 dB after 40 km single-mode fiber (SMF) transmission[12]. So in this Letter, we only discuss the upstream solution of the 100 G-PON. Since both the PAM-4 and EDB formats can relax the bandwidth requirement of the transceivers[13], a 10 G-class transmitter and receiver are used in the experiment. Figure 1 depicts the experimental setup. A commercially available 10 G-class EML is used as the 25 Gb/s upstream transmitter in the ONU. The data sequence is generated by a pulse-pattern generator (PPG, Keysight N4960A).
Sign up for Chinese Optics Letters TOC. Get the latest issue of Chinese Optics Letters delivered right to you!Sign up now
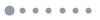
Figure 1.Experimental setup.
For 25 Gb/s PAM-4 format generation, two channels of 12.5 Gb/s data are used, followed by a PAM-4 encoder, and one channel is delayed by two pseudo-random bit sequence (PRBS) signals. At the OLT, we use an erbium-doped fiber amplifier (EDFA) to pre-amplify the upstream signal and then use an ODC with a dispersion tunability (II−VI Photonics PS3400) to compensate for the fiber dispersion from the differential reach. The group delay and calculated dispersion curves of the used ODC are shown in Ref. [10], which utilizes the same device used in this Letter. We also use an optical filter with a 4 nm bandwidth to suppress the amplified spontaneous emission (ASE) power from the EDFA, which can be replaced by a demux in symmetrical 100 G-PON systems. Then, we use a 10 G-class PIN (Conquer-KG-PR-10G) to detect the 25 Gb/s upstream signal. Due to lack of a real-time bit-error-rate (BER) tester for the EDB and PAM-4 formats, we use a digital storage oscilloscope (DSO) to capture the electrical signal and calculate the BER in Matlab. To simplify the calculation, the word length of the PRBS data is set at . Note that no digital signal processing algorithms are used to mitigate the system interference. The frequency response of the combined EML and PIN at an optical back-to-back (BtB) is shown in Fig. 2. It is noted that the 3 and 20 dB bandwidths of the system in the BtB case are about 8 and 14 GHz, respectively. Therefore, the system is more suitable for the EDB and PAM-4 formats to support 25 Gb/s per wavelength TDM-PON.
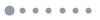
Figure 2.Frequency response of 10 G-class EML and PIN at BtB.
We conduct an experiment to investigate our proposed system architecture, as shown in Fig. 1. In consideration of the wavelength drift of the EMLs in the ONU side caused by the burst mode, we use a 4 nm bandpass filter to suppress the ASE power from the EDFA. So a 5 dB improvement of receiver sensitivity is achieved, as shown in Fig. 3. Thus, a 4 nm optical filter is always used in the following experiments. The BER for the 10 Gb/s data rate is also measured for reference. Compared to the transmission of the 10 Gb/s data rate, the PAM-4 is worse than 10 dB, which is caused by the requirement of device’s linearity for PAM-4 and limits the signal’s extinction ratio. Then, we evaluate the dispersion tolerance of 25 Gb/s PAM-4 signal on both the positive and negative dispersion values in the BtB case, as shown in Fig. 4. Obviously, the PAM-4 signal has much a stronger tolerance to negative dispersion, which is attributed to the positive chirp of the EML. But with the positive dispersion, the sensitivity of the PAM-4 is gradually worse than the BtB case. If the positive dispersion is more than 500 ps/nm, the BER performance cannot reach a sensitivity of . So PAM-4 is more sensitive to the positive dispersion of the fiber transmission. The BtB sensitivity (defined at a BER of ) varies with different dispersions and is shown in Fig. 5.
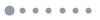
Figure 3.Receiver performance of 25 Gb/s PAM-4 with and without ASE filter and 10 Gb/s NRZ with ASE filter.
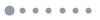
Figure 4.Upstream 25 Gb/s PAM-4 signal BER measurement with different positive (left) and negative (right) dispersion values in the BtB case.
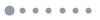
Figure 5.Upstream 25 Gb/s PAM-4 signal BtB sensitivity variations with dispersion.
With the positive dispersion increase from 0 to 600 ps/nm, the sensitivity is severely degraded, and even a BER cannot be achieved when the dispersion is higher than 500 ps/nm. But with negative dispersion from 0 to , we find the sensitivity varies in a small range between to . We discover that PAM-4 is more tolerant to the negative dispersion but is sensitive to the positive dispersion. For dispersion values between 0 and , the sensitivity remains unchanged. Considering both the positive and negative dispersion tolerances of the 25 Gb/s PAM-4 signal to support a 0–40 km differential reach, we decide to set the optimal dispersion value at in the OLT. Therefore, the residual dispersion values at the 0, 20, and 40 km reaches are , , and 180 ps/nm, respectively. For all the cases, the sensitivities remain at good values. The eye diagrams of the 25 Gb/s PAM-4 signal with and without the ODC in the BtB, 20, and 40 km fiber transmission cases are shown in Fig. 6. The eyes are significantly degraded after 20 and 40 km fiber transmission without the ODC; however, the eyes are clearly open for all the cases with the ODC, proving the feasibility of using a fixed ODC in the OLT to compensate for the dispersion from the differential reaches. Then, we calculate the BER of the 25 Gb/s PAM-4 signal for the different cases in Fig. 6, and the results are shown in Fig. 7. In all the reach cases, the sensitivity is around , and no significant transmission penalty is observed. Considering the EML output optical power of 6 dBm, a total 31 dB loss budget is achieved.
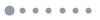
Figure 6.Eye diagrams of 25 Gb/s PAM-4 signal with and without dispersion compensation in BtB, 20, and 40 km fiber transmission cases.
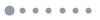
Figure 7.BER curves of 25 Gb/s PAM-4 signal in BtB, 20, 40 km fiber transmission cases.
Then, we evaluate the dispersion tolerance of the 25 Gb/s EDB signal. Similar to the PAM-4 case, we also place an ASE filter at the OLT side to suppress the EDFA noise. It is noted that the receiver sensitivity is improved about 2 dB, as shown in Fig. 8. So an ASE filter is also used in the following evaluation. We evaluate the dispersion tolerance of the 25 Gb/s EDB signal to both the positive and negative dispersion values in the BtB case, as shown in Fig. 9. To make a fair comparison with the PAM-4 case, the dispersion value is also tuned from to 600 ps/nm. Similar to the PAM-4 signal, the 25 Gb/s EDB signal also has a much stronger tolerance to negative dispersion. A BER cannot be achieved when the dispersion is higher than 400 ps/nm, and for the dispersion between 0 to , the sensitivity variation is within 4 dB. The BtB sensitivity as a function of the dispersion value for the 25 Gb/s EDB signal is shown in Fig. 10, where the variation trend is similar to the PAM-4 case. However, the sensitivity is better than the PAM-4 case, since the EDB format has only 3 levels and is more tolerant to the noise compared with the 4-level PAM-4 signal. Considering both the positive and negative dispersion tolerances of the 25 Gb/s EDB signal to support the 0–40 km differential reach, we set the optimal dispersion at in the OLT. The eye diagrams of the 25 Gb/s EDB signal with and without the ODC in the BtB, 20, and 40 km fiber transmission cases are shown in Fig. 11, and the corresponding BER results are shown in Fig. 12. The sensitivity in the BtB case with the ODC is the worst compared with the 20 and 40 km fiber transmission cases; however, the target is to achieve the best sensitivity at the longest distance due to the highest transmission loss, and the transmission dispersion penalty is within 2 dB. At a 40 km reach, the sensitivity is around , the same as the 20 km reach case. Considering the EML output optical power of 7.5 dBm, a total 39.5 dB loss budget is achieved, which is the record value for 25 Gb/s TDM-PON and would be a good candidate for 100 Gb/s PON. Note that the output power of the EML is higher for the EDB format, since it has lower linearity requirements on the transmitter compared with the PAM-4 format. Therefore, the EML can be biased at higher currents[14].
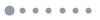
Figure 8.Receiver performances of 25 Gb/s EDB signal with and without ASE filter and 10 Gb/s NRZ with ASE filter.
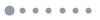
Figure 9.Upstream 25 Gb/s EDB signal BER measurement with different positive (left) and negative (right) dispersion values in the BtB case.
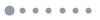
Figure 10.Upstream 25 Gb/s EDB signal BtB sensitivity variations with dispersion.
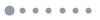
Figure 11.Eye diagrams of 25 Gb/s EDB signal with and without dispersion compensation in the BtB, 20, and 40 km fiber transmission cases.
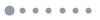
Figure 12.BER curves of 25 Gb/s EDB signal in the BtB, 20, and 40 km fiber transmission cases.
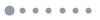
Figure 13.Upstream burst-mode timing sequences at (a) 1000 and (b) 100 ns/div.
As for the two upstream schemes, 39.5 and 31 dB loss budgets for the 25 Gb/s EDB and PAM-4 signals have been achieved, respectively, and both support the 0–40 km differential reach. So the EDB performance is better than that of the PAM-4. The main advantages of the EDB and PAM-4 schemes are that the system bandwidth requirement is relaxed and both of them are more CD tolerant compared with the NRZ format. So we can use relatively low-bandwidth optical devices to support high-speed transmissions. The PAM-4 format requires a linear transceiver, which limits the optical signal extinction ratio and is more challenging for the upstream burst-mode receiver[15]. As for the EDB format, only the demodulation may need a high-speed duobinary-to-binary conversion circuit, which may increase the system cost. But the increased cost of the OLT can be shared by all users. So the EDB format using 10 G-class EML and PIN for the upstream scheme would be a better solution. Figure 13 shows the burst-mode timing sequences of the upstream scheme. We assume a preamble that accommodates laser turn-on and residual burst settling effects.
In conclusion, we propose to use an ODC with negative dispersion in an OLT to support a 0–40 km differential reach for 25 Gb/s upstream signals. The optimal dispersion value is evaluated for both PAM-4 and EDB formats to achieve the highest loss budget and the lowest transmission dispersion penalty. The maximal loss budget of 39.5 dB can be achieved for the EDB format, which is the record value for 25 Gb/s TDM-PON. Additionally, the ODC can support multi-channel operations. Therefore, the EML-based EDB format in the ONU combined with an ODC in the OLT will be an attractive solution for the upstream direction in symmetrical 100 G-PONs.
References
[1] H. Zhang, S. Fu, J. Man, W. Chen, X. Song, L. Zeng. Proceedings of OFC(2014).
[2] V. Houstma, D. V. Veen. Proceedings of ECOC, 1(2015).
[3] J. Wei, N. Eiselt, H. Griesser, K. Grobe, M. Eiselt, J. J. Vegas-Olmos, I. T. Monroy, J.-P. Elbers. Proceedings of ECOC(2015).
[4] Z. Li, L. Yi, X. Wang, W. Hu. Opt. Express, 23, 20249(2015).
[5] V. Houtsma, D. van Veen, A. Gnauck, P. Iannone. Proceedings of OFC(2015).
[6] D. van Veen, V. E. Houtsma, P. Winzer, P. Vetter. Proceedings of ECOC(2012).
[7] Z. Ye, S. Li, N. Cheng, X. Liu. Proceedings of ECOC(2015).
[8] M. Tao, L. Zhou, S. Yao, D. Zou, S. Li, H. Lin, X. Liu. Proceedings of OFC(2016).
[9] Z. Zhang, X. Jiang, J. Wang, X. Chen, L. Wang. Chin. Opt. Lett., 13, 020603(2015).
[10] Y. Guo, S. Zhu, G. Kuang, Y. Yin, Y. Gao, D. Zhang, X. Liu. Proceedings of OFC(2014).
[11] L. Yi, X. Wang, Z. Li, J. Huang, J. Han, W. Hu. Opt. Express, 23, 7971(2015).
[12] L. Yi, H. Ji, Z. Li, X. Li, C. Li, Q. Yang, L. Xue, X. Wang, S. Wang, Y. Yang, J. Xu, S. Yu, W. Hu. Proceedings of ECOC(2016).
[13] Z. Li, L. Yi, H. Ji, W. Hu. Opt. Express, 24, 12941(2016).
[14] L. Han, S. Liang, H. Zhu, W. Wang. Chin. Opt. Lett., 13, 081301(2015).
[15] D. van Veen, V. Houtsma. Proceedings of OFC(2016).