Abstract
We report a hybrid femtosecond laser system based on a femtosecond Yb-doped fiber laser and a Yb-doped potassium gadolinium tungstate (Yb:KGW) regenerative amplifier. To match the central wavelength of the seed source, a Yb:KGW crystal is used in the regenerative amplifier for polarization. We study and optimize the dynamics of nonlinear amplification to alleviate the gain narrowing effect. With optimization, the system can output 270 fs pulses with pulse energy at a 60 kHz repetition rate.In recent years, femtosecond lasers with high pulse energy have gained significant attention in many research and industrial applications. Ultrafast fiber and solid-state laser amplification systems are both potentially important techniques for producing high-power, high-energy femtosecond laser sources[1–3]. The fiber scheme offers major advantages in terms of compactness, lack of misalignment, and environmental insensitivity[4–7] compared with mode-locked solid-state laser[8], while amplifiers with bulk gain media extract intrinsically high output energies[9,10]. Thus, a hybrid system with a fiber seed source and a solid-state amplifier can inherit the advantages of both. The regenerative amplification scheme, which offers a gain of several orders, can be used to amplify a nanojoule (nJ)-level fiber laser source to tens of microjoules () at multi-kilohertz repetition rates[11,12]. The regenerative amplifier combined with the fiber seed source is an alternative that offers high-energy output with a stable and compact structure.
For hybrid femtosecond laser amplification systems, spectral matching between fiber laser sources and bulk media in amplifiers is an essential issue. Among various materials, Yb-doped rare-earth potassium tungstates [Yb-doped potassium gadolinium tungstate (Yb:KGW), Yb-doped potassium yttrium tungstate (Yb:KYW)] are promising for high-emission cross sections, broad gain bandwidth, and good thermal conductivity[13]. But, optical properties of Yb:tungstates exhibit anisotropy for different polarizations, owing to their crystalline structure[14]. Most previous works with Yb:KGW/Yb:KYW crystals have concentrated upon laser amplification for -polarized modes, which show larger gain coefficients than -polarized modes[15–17]. Unfortunately, the gain spectrum of Yb:KGW crystals with polarization does not match the spectrum of a 1040 nm femtosecond fiber laser, which is usually used as a seed source. In order to overcome this problem, a regenerative amplifier with an electric field parallel to the axis is applied in this Letter.
The gain bandwidth of Yb:KGW is , which can support sub-200-fs pulses. However, pulses output from Yb:KGW regenerative amplifiers are normally compressed to 300–500 fs due to gain narrowing in amplifiers[18]. To obtain shorter pulses, several methods have been developed to extend the output spectrum. Using dual Yb:KGW crystals with two different optical axes ( and ), the output spectra are broadened to [19,20]. However, this makes the systems rather complex and bulky. Another strategy is nonlinear amplification, which broadens spectra by self-phase modulation (SPM) to alleviate the gain narrowing effect[21,22].
Sign up for Chinese Optics Letters TOC. Get the latest issue of Chinese Optics Letters delivered right to you!Sign up now
In this Letter, we demonstrate a hybrid amplification system based on a femtosecond Yb-doped fiber laser and a Yb:KGW regenerative amplifier. For central-wavelength matching, Yb:KGW is utilized for polarization. To obtain short pulses, nonlinear spectral broadening is induced by SPM in the amplification. Meanwhile, the nonlinear regenerative amplification dynamics of different round trips is investigated at the optimal gate time. By optimizing the nonlinear process in the amplifier, the system can output 270 fs pulses with pulse energies after compression. To the best of our knowledge, this is the shortest pulse that has been reported for -polarized-laser output of a Yb:KGW crystal.
The experimental setup is depicted in Fig. 1. The hybrid femtosecond amplifier consists of an oscillator, a pre-chirper, a pre-amplifier, an acousto-optic modulator (AOM), a Yb:KGW regenerative amplifier, and a grating compressor. The home-made oscillator is a mode-locked -doped fiber laser operating in the all-normal-dispersion (ANDi) regime. The oscillator delivers 4.5 ps pulses with a 138 mW average power at a 60 MHz repetition rate, with excellent power stability of less than 0.2% root mean square (RMS). The output spectrum is centered at 1038 nm with a 16 nm bandwidth, as shown in Fig. 2(a). With a 1200 line/mm grating pair, pulses from the fiber laser oscillator can be dechirped to 182 fs, and the autocorrelation trace is shown in Fig. 2(b). To obtain the shortest compressed pulse duration at the output, the pre-chirp of seed pulses is optimized. For the optimal case, the gratings are separated by a 2.1 cm perpendicular distance, providing group velocity dispersion (GVD). Pulses are compressed to 280 fs with positive chirp [Fig. 2(b), blue].
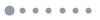
Figure 1.Schematic of hybrid fiber/solid-state femtosecond laser amplifier. PCF, photonic crystal fiber; TFP, thin film polarizer; PC, Pockels cell; DM, dichroic mirror; LD, laser diode.
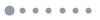
Figure 2.(a) Spectra of the oscillator (blue) and the PCF pre-amplifier (black). (b) Autocorrelation traces of the dechirped pulse from the oscillator (red), the pre-chirped pulse from the oscillator (blue), and the pre-chirped pulse from the pre-amplifier (black).
The gain medium of the pre-amplifier is a 1-m-long single-polarization -doped large-mode-area double-cladding photonic crystal fiber. This fiber amplifier is used to compensate for the loss of the pre-chirper and AOM and provide adequate seed pulse energy. The pulses in the pre-amplifier are stretched to 466 fs, as shown in Fig. 2(b). An AOM is applied to reduce the repetition rate of the seed pulses from 60 MHz to 60 kHz, and the pulse energy is after AOM.
In the main amplifier, a double beta-barium borate (BBO) Pockels cell, a quarter-wave plate, and a thin film polarizer compose an optical gate to trap seed pulses and extract output pulses. The gain medium is a 5-at %-doped Yb:KGW crystal cut along the axis with a 4 mm thickness. The pump absorption efficiency of this Yb:KGW crystal is . The pump source is a 25 W 981 nm diode laser with a pigtail fiber ( core diameter). To avoid aberrations of the pump beam, two aspherical lenses with focal lengths of 30 and 60 mm are utilized and provide a magnification factor of 1:2 for mode matching between the pump and laser. As shown in Fig. 1, a half-wave plate is placed in the cavity. The -polarized and -polarized modes can be conveniently switched by rotating the plate. The entire length of this cavity is 1.5 m, corresponding to a round-trip time of 10 ns. Finally, the extracted pulse is compressed by 1600 lines/mm transmission gratings with 72% efficiency.
First, we study the amplification performance of lasers parallel to the axis. In the -switching regime, the average output power is 2.3 W at a 60 kHz repetition rate with an output spectrum centered at 1038 nm. For the amplification scheme, the build-up time before reaching saturation is 1000 ns, corresponding to 100 round trips [see Fig. 3(a), blue curve]. Due to the short duration of seed pulses, the SPM is obvious when pulses propagate in the cavity, and it broadens the output spectrum. The output 10 dB spectral bandwidth is a function of the round-trip number, as shown in Fig. 3(a). The evolution of output spectra versus round trip is also illustrated in Fig. 3(b). Output spectra rapidly broaden between round trips 20 and 50. The gain narrowing effect is negligible, since the gain is not very high. Because the seed spectrum shows a “cat-ear” shape, and the cavity dispersion is positive, the peaks on two edges of the spectrum are much higher than others. The typical SPM-induced broadening in the positive-dispersion medium results in the steepness of the pulse edges, as shown in Fig. 3(c). The maximum 10 dB bandwidth obtained at 500 ns is . After compression, the pulse duration is 260 fs, and the autocorrelation trace (red curve) is shown in Fig. 3(d). More than 90% of the total energy is concentrated in the main pulse.
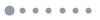
Figure 3.(a) 10 dB spectral bandwidth (left) and average power (right) versus round-trip number. (b) Evolution of the spectrum during amplification. (c) Output pulse spectra and (d) autocorrelation traces of dechirped pulses with an optical gate time of 300 ns (green), 400 ns (blue), 500 ns (red), and 1000 ns (black).
Between round trips 50 and 100, the average output power still increases. The maximum average power is 1.8 W for 100 round trips, corresponding to a pulse energy. In this period, gain shaping is more obvious due to higher gain. Thus, the energy of the spectral component near 1038 nm (gain center of Yb:KGW for the polarization) substantially increases, as shown in Fig. 3(b). As a result, the gain narrowing effect dominates over the nonlinear effects, and the spectral bandwidth slightly decreases. When the gate time is 1000 ns, the output spectrum shows a bandwidth of with 1039 nm central wavelength [as shown in Fig. 3(c)]. After compression, the pulse duration is 270 fs, and the autocorrelation trace is shown in Fig. 3(d). The dechirped pulse energy is . Compared with pulses generated at a 500 ns gate time, the pulses show a higher pedestal. In this stage, SPM is detrimental, and the pulse quality is degraded by an uncompensated nonlinear phase shift.
As depicted in Fig. 4, the output energy of the hybrid amplifier is at a repetition rate of 60–240 kHz. The corresponding average power is between 1.8 and 2.6 W. The beam profile of the amplified pulse is measured by a CCD camera, as shown in the inset of Fig. 4. As a result of beam degradation from the AOM and the thermal lensing effect, the output beam of the main amplifier is a little elliptical, but it remains a fundamental mode.
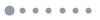
Figure 4.Average output power (left) and pulse energy (right) versus different repetition rates and beam profiles at 60 kHz (inset).
For comparison, we also demonstrate regenerative amplification along the axis. In the -switching regime, the spectrum is centered at 1029 nm with a 4.5 nm bandwidth. For the amplification scheme, the build-up time at the saturation point decreases to 400 ns, since the gain coefficient for the polarization is higher. However, the extracted pulse energy is only half of that when is polarized as a result of mismatch between the gain media and the seed source. The 10 dB output bandwidth is 14.5 nm, and the central wavelength is 1030 nm [as shown in Fig. 5(a), red curve]. The compressed pulse duration is . Figure 5(b) shows a comparison of autocorrelation traces for two orthogonal polarizations. The pulse of the -axis amplification scheme shows longer duration and poor temporal quality. During amplification, the short-wavelength edge obtains more gain, since the gain center of axis is at 1029 nm. Unfortunately, for the ANDi mode-locked fiber laser, it is typical for the phase at the edges of seed pulses to be nonlinear, while that in the middle of the pulses is linear. As a result, the short-wavelength edge of the seed pulse with nonlinear phase is amplified, inducing an obvious pedestal after compression. Therefore, it is beneficial for a hybrid femtosecond laser system to operate along the axis of Yb:KGW.
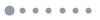
Figure 5.(a) Spectra of injected seed source (black) and amplified pulses for polarization (blue) and polarization (red). (b) Autocorrelation traces of output pulses for polarization (blue) and polarization (red).
In conclusion, we demonstrate a hybrid nonlinear regenerative amplifier operating with two orthogonal polarizations, respectively. For polarization, 350 fs pulses with pulse energy are generated, whereas for polarization, pulse energy is as high as , and the pulse duration is 270 fs. Gain narrowing is suppressed by introducing SPM during amplification. Moreover, pre-chirping is optimized to control the nonlinear amplification for the shortest output pulse duration. The 16 nm output spectral bandwidth is achieved during amplification, supporting a 270 fs dechirped pulse duration. To the best of our knowledge, this is the shortest pulse ever amplified along the axis of a Yb:KGW crystal. This hybrid scheme provides a compact and robust femtosecond laser architecture for various requirements in the industrial and scientific fields.
References
[1] M. Kienel, M. Müller, A. Klenke, J. Limpert, A. Tünnermann. Opt. Lett., 41, 3343(2016).
[2] Y. Chen, C. Zhao, S. Chen, J. Du, P. Tang, G. Jiang, H. Zhang, S. Wen, D. Tang. IEEE J. Sel. Top. Quantum Electron., 20, 315(2014).
[3] B. Dannecker, J.-P. Negel, A. Loescher, P. Oldorf, S. Reichel, R. Peters, T. Graf, M. A. Ahmed. Opt. Commun., 429, 180(2018).
[4] A. Chong, J. Buckley, W. Renninger, F. Wise. Opt. Express, 14, 10095(2006).
[5] M. E. Fermann, I. Hartl. Nat. Photon., 7, 868(2013).
[6] L. Zhao, D. Tang, X. Wu, H. Zhang. Opt. Lett., 35, 2756(2010).
[7] J. Shi, Y. Li, S. Y. Gao, Y. L. Pan, G. M. Wang, R. Y. Ji, W. H. Zhou. Chin. Opt. Lett., 16, 121404(2018).
[8] J. Ma, S. Lu, Z. Guo, X. Xu, H. Zhang, D. Tang, D. Fan. Opt. Express, 23, 22643(2015).
[9] Z. T. Fan, Z. B. Gan, X. Y. Liang, L. H. Yu, W. Q. Li, Z. Guo, X. L. Yuan, H. Cao, P. Huang, R. X. Li, Z. Z. Xu. Chin. Opt. Lett., 15, 061401(2017).
[10] S. Biswal, J. Itatani, J. Nees, G. Mourou. IEEE J. Sel. Top. Quantum Electron., 4, 421(1998).
[11] S. R. Bowman, S. P. O’Connor, S. Biswal. IEEE J. Quantum Electron., 41, 1510(2005).
[12] C. L. Chang, P. Krogen, K. H. Hong, L. E. Zapata, J. Moses, A. L. Calendron, H. Liang, C. J. Lai, G. J. Stein, P. D. Keathley, G. Laurent, F. X. Kärtner. Opt. Express, 23, 10132(2015).
[13] C. Hönninger, R. Paschotta, M. Graf, F. Morier-Genoud, G. Zhang, M. Moser, S. Biswal, J. Nees, A. Braun, G. A. Mourou, I. Johannsen, A. Giesen, W. Seeber, U. Keller. Appl. Phys. B, 69, 3(1999).
[14] H. Zhao, A. Major. Opt. Express, 22, 26651(2014).
[15] A. Beyertt, D. Muller, D. Nickel, A. GiesenAdvanced Solid-State Photonics 2004, WA5.
[16] A. Calendron, K. Wentsch, J. Meier, M. J. Lederer. Conference on Lasers and Electro-Optics, CFD2(2009).
[17] K. Ogawa, Y. Akahane, M. Aoyama, K. Tsuji, S. Tokita, J. Kawanaka, H. Nishioka, K. Yamakawa. Opt. Express, 15, 8598(2007).
[18] H.-H. Liu, J. Nees, G. Mourou. Opt. Lett., 27, 722(2002).
[19] A. Buettner, U. Buenting, D. Wandt, J. Neumann, D. Kracht. Opt. Express, 18, 21973(2010).
[20] G. H. Kim, J. Yang, S. A. Chizhov, E. G. Sall, A. V. Kulik, V. E. Yashin, D. S. Lee, U. Kang. Opt. Express, 20, 3434(2012).
[21] J. Pouysegur, M. Delaigue, C. Hönninger, P. Georges, F. Druon, E. Mottay. Opt. Express, 22, 9414(2014).
[22] J. Pouysegur, M. Delaigue, C. Hönninger, Y. Zaouter, P. Georges, F. Druon, E. Mottay. IEEE J. Sel. Top. Quantum Electron., 21, 212(2015).