Abstract
The continuous wave (CW) and passively -switched (PQS) performances of diode-pumped (Nd:LaGGG) at 1.33 μm are achieved for the first time to our knowledge. The maximum CW output power of 5.1 W is obtained with the optical-optical conversion efficiency of 25.3% and the slope efficiency of 26.6%. In the PQS operation, by using the crystal as the saturable absorber, the maximum average output power, shortest pulse width, largest pulse energy, and highest peak power are measured to be 1.1 W, 27.54 ns, 75.78 μJ, and 2.44 kW, respectively.Diode-pumped solid-state lasers possess the merits of compactness, high efficiency, reliability, and good beam quality that have enabled them to have extensive applications in industry, medicine, and scientific studies[1]. Considerable efforts have been devoted to search for good solid-state gain media in which (Nd:GGG) has been proved to be an excellent candidate for laser applications owing to its good mechanical properties, large thermal conductivity, and high damage threshold[2,3]. As an isomorph of GGG crystal, a Nd:LaGGG crystal with a doping level of 1 at. % and a concentration of 1.6 at. % has been grown by the Cz method[4]. In comparison with the Nd:GGG crystal, the Nd:LaGGG crystal should have a lower melting temperature and a higher segregation coefficient owing to the larger radius of ions than that of ions[5], which would benefit the crystal growth. Furthermore, the Nd:LaGGG crystal can possess wider inhomogeneous broadened spectra than that of Nd:GGG crystal because of the multicenter distribution of ions and more complex structure of the host material[4], which would be favorable for mode-locking operation. The crystal growth, the thermal and spectral properties, as well as the laser properties at 1062 nm of Nd:LaGGG crystal have been investigated[4,6]. However, to our knowledge, no work on 1.3 μm Nd:LaGGG lasers has been reported yet.
The continuous wave (CW) and passively -switched (PQS) laser properties of some Nd-doped gallium-based garnets at 1.3 μm have been widely studied, as shown in Table 1[7–12], in which the Nd:LGGG crystal doped with 0.66 at. % ions owns the highest output power on CW (3.7 W) and PQS (0.75 W) operation. Concerning the saturable absorber applied in this work, we selected the crystal because it has good physical and optical performance around 1.3 μm in comparison with the co-doped crystals and the semiconductor saturable absorber mirror (SESAM)[9].
| CW | PQS |
---|
Laser properties | Pout (W) | ηopt | ηp | Pave (W) | ΔT (ns) | Ppeak (kW) | E (μJ) | Ref. |
---|
Nd:GGG | 2.08 | 19.0% | 20.5% | 0.46 | 19.0 | 0.6 | 11.8 | [7] |
| 1.50 | 19.4% | 21.5% | 0.18 | 16.4 | 1.3 | 21.4 | [8] |
Nd:GAGG | 2.45 | 21.8% | 23.3% | 0.33 | 18.2 | 2.0 | 36.3 | [9] |
Nd:LGGG | 0.93 | 8.5% | 10.4% | 0.17 | 15.6 | 1.1 | 16.7 | [10] |
| 3.70 | 19.6% | 20.9% | 0.75 | 25.9 | 1.7 | 43.6 | [11] |
Nd:YGG | 3.09 | 16.7% | 21.3% | – | – | – | – | [12] |
Table 1. CW and PQS Laser Properties of Some Nd-doped Gallium-based Garnets at 1.3 μm
In this Letter, we report CW and PQS laser operations at 1.33 μm with the Nd:LaGGG crystal. Under the absorbed pump power of 20.16 W, the highest CW output power of 5.1 W was achieved with the optical-optical conversion efficiency of 25.3% and the slope efficiency of 26.6%. By using crystal as the saturable absorber, we demonstrated the PQS Nd:LaGGG laser with the maximum average output power of 1.1 W.
Sign up for Chinese Optics Letters TOC. Get the latest issue of Chinese Optics Letters delivered right to you!Sign up now
The experimental arrangement of the diode-pumped -switched Nd:LaGGG crystal at 1.33 μm is shown schematically in Fig. 1. The pump source was a fiber-coupled 808 nm diode laser with a core diameter of 0.6 mm and numerical aperture of 0.22. Its radiation was coupled into the laser crystal by a focusing optical system with a 25 mm focal length and a ratio of . The Nd:LaGGG crystal was cut along the direction with dimensions of . It was wrapped with indium foil and mounted in copped block cooled by water at a temperature of 20°C. The input concave mirror M1 with the radius of curvature 800 mm was antireflection (AR) coated at 808 nm on the plane side, high reflection (HR, ) at 1330 nm, and high transmission (HT, ) at 808 nm on the concave side. The output mirror M2 had a coating with different transmissions (, 8%, and 15%) at 1330 nm and HT at 1062 nm to suppress the oscillation of 1.06 μm laser. The saturable absorber with the dimensions of was placed next to output mirror M2 to realize the -switching operation. Its initial transmission at 1.3 μm was measured to be about 95%. The CW and PQS laser output power was detected by the laser power meter (Fieldmax II, coherent). The laser pulse signal was recorded by a Tektronix DPO7104 digital oscilloscope (1 GHz bandwidth, 5 Gs/s sampling rate) and a photodetector (New Focus, model 1611).
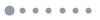
Figure 1.Experimental arrangement of diode-pumped Nd:LaGGG crystal at 1.33 μm.
As a fundamental optical parameter of laser crystals, the stimulated emission cross section () of transition around 1.33 μm for Nd:LaGGG crystal was calculated by the method at room temperature[13]. Figure 2 shows the result together with that of the Nd:LGGG crystal () for comparison[4]. Since Nd:LaGGG has a smaller () and larger excited state fluorescence lifetime (243 μs) than those of Nd:LGGG[14] ( and 220 μs, respectively), Nd:LaGGG crystal should have better -switching laser performance in the 1.33 μm region.
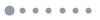
Figure 2.Room-temperature stimulated emission cross section of Nd:LGGG () and Nd:LaGGG at around 1.3 μm.
The relationship between the thermal focal length and the incident pump power was studied first, which could be expressed as [9], where is the pump beam radius in the laser medium and is a constant obtained by fitting the experimental data with the above formula. The value of was measured by referring the methods adopted in Ref. [15]. The output power was optimized near the pump threshold at a given cavity length. While the pump power increased to a critical value, the strong thermal lensing effect caused the laser cavity to become unstable, which would result in a rapid decrease of the CW output power. The corresponding cavity length could be regarded as the approximate thermal focal length under the corresponding critical pump power. For Nd:LaGGG crystal, the relationship between the thermal focal length and absorbed pump power with determined to be was shown in Fig. 3. The thermal focal length decreased with the increase of absorbed pump power, which indicates that a shorter cavity length would be suitable for laser operations. Therefore, considering the severe thermal effect, CW operations of Nd:LaGGG crystal at 1.33 μm were first carried out by removing from the cavity and optimizing the cavity length to be 15 mm.
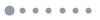
Figure 3.Relationship between the thermal focal length and absorbed pump power.
The absorption efficiency of the Nd:LaGGG crystal at 808 nm was measured to be 90.24%. Figure 4 shows the output power of the CW Nd:LaGGG laser at 1.33 μm with different output couplers of , 8%, and 15%. The threshold pump power () were determined to be 0.36, 0.97, and 2.2 W, respectively. The maximum output power values of 4.91, 5.1, and 3.58 W were obtained under the absorbed pump power of 20.16 W, with the output coupler of , respectively. The corresponding optical-to-optical conversion efficiency for the three situations were respectively calculated to be 24.4%, 25.3%, and 17.8%, while the slope efficiency values were obtained to be 24.8%, 26.6%, and 19.9%. Compared with the Nd:LGGG crystal (as shown in Table 1), not only for the highest output power but for the optical conversion efficiency, the Nd:LaGGG crystal has a better laser performance at 1.33 μm, which indicates that the Nd:LaGGG crystal is of the high quality for the laser output.
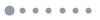
Figure 4.CW output power versus absorbed pump power.
For the PQS regime, it is of great importance that the saturation that occurs in the saturable absorber should be earlier than that in the gain medium, named the second threshold criteria[9]. The smaller emission cross section of the Nd:LaGGG crystal () at 1.33 μm makes the second threshold criteria be easily satisfied with the saturable absorber of . The average output power versus the absorbed pump power is shown in Fig. 5 with the output couplers of , 8%, and 15%, respectively. The corresponding passive -switching thresholds were 2.51, 3.11, and 4.78 W, respectively. Under the absorbed pump power of 12.84 W, the maximum output power of 1.1 W was obtained with . With the increase of the absorbed pump power being higher than 12.84 W, the output power decreased sharply at and 8%, while the output power gradually increased at for higher transmittance leading to a lower thermal effect compared with the other two output couplers. Compared with the passive -switching performance of Nd-doped gallium-based garnets in Table 1, the output power of the Nd:LaGGG laser at 1.3 μm was the highest. In addition, the rms power stability was also estimated by a power meter with output power of 1.1 W and frequency of 10 Hz, which was determined to be 1.2% for 1 h operation.
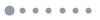
Figure 5.-switched output power versus absorbed pump power.
The variation of the repetition and pulse width versus the absorbed pump power is shown in Fig. 6, in which the repetition rate increased with increasing pump power at all three different output couplers. When increasing the pump power, the density of the inverse population gathering on the excited state enlarged correspondingly, causing the net gain to efficiently add and making the time of the gain exceed the threshold shorted. That is to say, the period of the -switching is shorted and the repetition rate is increasing accordingly. The maximum repetition rate of 21.56, 20.45, and 13.66 kHz was obtained at , 8%, and 15%, respectively.
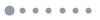
Figure 6.Variation of repetition and pulse width versus the absorbed pump power.
Under the absorbed pump power of 14.30 W, the shortest pulse width of 27.54 ns was obtained at . We got the shortest pulse width of 33.88 ns at and 31.08 ns at separately under the absorbed pump power of 9.75 W. The noise-signal ratio was estimated to be 38 dB with the average output power of 1.1 W. The maximum single-pulse energy and peak power was calculated to be 75.78 μJ at with the absorbed pump power of 9.75 W, and 2.44 kW at with the absorbed pump power of 14.30 W, respectively. The shortest pulse width profiles, along with the corresponding pulse train, are shown in Fig. 7.
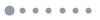
Figure 7.Pulse train with the repetition rate of 13.66 kHz. The upper left inset shows the pulse profile with the pulse width of 27.54 ns.
The 1.33 μm CW and PQS performances of Nd:LaGGG crystal with the doping level of 1 at. % and 1.6 at. % inside are reported for the first time to our knowledge. A maximum CW output power of 5.1 W is obtained under an absorbed pump power of 20.16 W, giving a maximum slope efficiency of 25.3% and an optical conversion efficiency of 26.6%. Using a crystal as the saturable absorber, the maximum average output power of 1.1 W is obtained with . The minimum pulse width of 27.54 ns, pulse peak power of 2.44 kW, and maximum single pulse energy of 75.78 μJ are achieved at when the crystal with the initial transmission of 95% is used. All of the results indicate that the Nd:LaGGG crystal should be a promising laser material for CW and PQS laser operation at 1.33 μm.
References
[1] Y. Kunio, Y. Hidetsugu, K. Yoshiaki. IEEE J. Quantum Electron., 24, 1118(1988).
[2] Z. Zhao, B. Jiang, Y. Zhang, Y. Liu, X. Xu, P. Song, X. Wang, J. Xu. Chin. Opt. Lett., 3, 163(2005).
[3] C. Zuo, J. Hou, B. Zhang, J. He. Chin. Opt. Lett., 13, 021401(2015).
[4] X. Fu, Z. Jia, Y. Li, D. Yuan, C. Dong, X. Tao. Opt. Mater. Express, 2, 1242(2012).
[5] B. Cockayne, D. Gasson, D. Findlay, D. Goodwin, R. Clay. J. Phys. Chem. Solids, 29, 905(1968).
[6] H. Yang, X. W. Fu, Z. T. Jia, J. L. He, X. Q. Yang, B. T. Zhang, R. H. Wang, X. M. Liu, J. Hou, F. Lou, Z. W. Wang, Y. Yang. Laser Phys. Lett., 9, 704(2012).
[7] C. Zuo, B. Zhang, J. He, X. Dong, K. Yang, H. Huang, J. Xu, S. Zhao, C. Dong, X. Tao. Opt. Mater., 31, 976(2009).
[8] C. Zuo, B. Zhang, J. He, X. Dong, J. Yang, H. Huang, J. Xu, S. Zhao, C. Dong, X. Tao. Appl. Phys. B Lasers Opt., 95, 75(2009).
[9] B. Zhang, J. Yang, J. He, H. Huang, S. Liu, J. Xu, F. Liu, Y. Zhi. Opt. Express, 18, 12052(2010).
[10] L. Shande, J. Zhitai, H. Jingliang, Z. Baitao, Y. Xiuqin, L. Fengqin, X. Jinlong, T. Xu-Tang. IEEE Photonics Technol. Lett., 24, 146(2012).
[11] Z. Wang, X. Fu, J. He, Z. Jia, B. Zhang, H. Yang, R. Wang, X. Liu, X. Tao. Laser Phys. Lett., 10, 055005(2013).
[12] Y. Li, J. Li, X. Liu, J. Wu, X. Zhang. Laser Phys., 22, 531(2012).
[13] B. Aull, H. Jenssen. IEEE J. Quantum Electron., 18, 925(1982).
[14] X. Fu, Z. Jia, H. Yang, Y. Li, D. Yuan, B. Zhang, C. Dong, J. He, X. Tao. Laser Phys. Lett., 9, 833(2012).
[15] Y. Chang, Y. Huang, K. Su, Y. Chen. Opt. Express, 16, 21155(2008).