Ken T. Shimizu1、*, Marcel Böhmer2, Daniel Estrada1, Sumit Gangwal1, Stefan Grabowski3, Helmut Bechtel3, Edward Kang1, Kenneth J. Vampola1, Danielle Chamberlin1, Oleg B. Shchekin1, and Jyoti Bhardwaj1
Abstract
We demonstrate the first commercial production–ready white light-emitting diodes (LEDs) for the general illumination market with red colloidal quantum dots (QDs) applied in an on–chip configuration. We show the red QDs with tunable peak emission and narrow full width at half-maximum in combination with a conventional phosphor material can lead to LED conversion efficiency improvements of 5% to 15% over commercial phosphor based LEDs at correlated color temperatures (CCTs) ranging from 5000 to 2700 K. Furthermore, the challenges associated with reliability under high temperature, high blue flux intensity, and high humidity operation have been overcome to meet consumer market requirements. Finally, a demonstrator lamp at 3000 K color temperature and 90 color rendering index (CRI) with QD based LEDs show a larger efficiency gain up to 17%, attributed to the reduced blue LED droop from the lower drive current and the lower heat sink temperature when compared to a standard phosphor based LED lamp output.1. INTRODUCTION
Quantum dots (QDs) have been described as artificial atoms due to the quantized electronic states arising from the exciton quantum confinement [1]. QDs demonstrate a size-dependent tunable bandgap that can span the entire visible spectrum, narrow full width at half-maximum (FWHM) owing to the direct transition from the bandgap edge, high absorption cross section in the blue color region appropriate for InGaN light-emitting diode (LED) excitation, fast radiative excited state lifetime, and high quantum efficiency approaching unity at room temperature [2–5].
The first commercial applications of QDs were used as in vitro fluorescent labels substituting for organic dyes due to their photo-oxidation robustness and singular excitation for the various emission wavelengths [6,7]. More recently, the use of QDs for high gamut liquid crystal displays has seen wide adoption in consumer products as a replacement for phosphors in high gamut standard and ultrahigh definition (UHD) televisions [8]. In these implementations, the QDs are either embedded in a polymer film with oxygen barrier coatings applied to both sides or confined in an environmentally sealed glass optic. The QD film is placed remotely from the blue LED in the screen of the display and optically excited by blue LEDs in a separate light bar component. The QD optic is placed above the LED light bar [9]. In both cases, this allows the QDs to operate with blue light intensities at or below [10]. To date, QDs have failed as a drop-in solution into the LED package because the LED operating temperature and blue flux intensities caused dramatic thermal quenching and fast photodegradation [11–13]. Nevertheless, an on-chip phosphor application in silicone is preferred over remote phosphor configurations as it provides the lowest cost and lowest complexity solution for LED use in lighting and display applications.
We report on the on-chip application of QDs where the QD temperature exceeds 100°C and the blue flux intensity reaches in midpower LED packages that demonstrate the necessary reliability requirements for commercial use and provide double digit performance improvements. As with other narrow red emitters, QDs offer the greatest benefit when producing high color rendering index (CRI) light to bring LED lamps closer to the high color quality of incandescent light sources [14]. The following 90 CRI LEDs are compared: red QD based LEDs, red nitride based phosphor LEDs, and a hybrid LED module mixing a red AlInGaP LED and a phosphor converted off-white LED.
Sign up for Photonics Research TOC. Get the latest issue of Photonics Research delivered right to you!Sign up now
2. EXPERIMENTAL
For all experiments described in this paper, engineering builds of LUXEON 3535L HE Plus LEDs were used to validate the performance and reliability as a representative product platform.
Figure 1(a) shows an image of this industry standard midpower LED, and Fig. 1(b) shows the schematic top and side views where two LED chips are attached and wire bonded in parallel to a molded lead frame package. The package dimensions are , the emitting surface diameter is 3 mm, and the internal volume for the phosphor slurry is approximately 3 μL [15]. The manufacturing procedure includes standard mixing of phosphor and QD material into commercial phenyl based silicone and dispensing into the packages. Red (625 nm) QDs in solvent-free dry powder form with FWHM at 25°C (40 nm at 100°C) were received from Pacific Light Technologies and processed under similar conditions to other phosphor materials. The QD and silicone mixture was dispensed into the LED package and cured for 4 h at 150°C in an oven. The LEDs were solder attached following JEDEC J–STD–020D reflow procedures using tin–silver–copper (SAC) solder to a starboard for measuring light output and stress testing. Red nitride phosphor based LEDs for the same correlated color temperatures (CCTs) and CRIs as the QD based LEDs were fabricated in a similar fashion for direct comparison of performance and reliability. Drive conditions can vary based on the end application. Here we showcase two real-world operating condition examples: 60–75 mA at 60°C for downlight and troffer applications to 200–400 mA at 85°C–105°C for retrofit light bulbs.
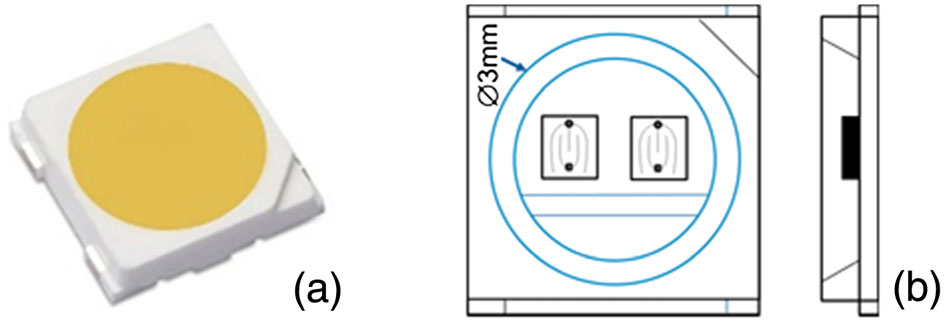
Figure 1.LUXEON 3535L HE Plus lead frame LED package was used for integrating and testing QDs in silicone resins.
3. RESULTS AND DISCUSSION
A. Modeling
Table 1 shows the theoretical modeling that compares the impact of the peak FWHM for red and green emitters to the lumen equivalent (LE) or lumen efficacy of radiation of white LED spectra normalized to the corner case of 110 nm green and 90 nm red. This is a proprietary spectrum based model that propagates light from the LED die through the phosphors and/or QDs and includes interactions with the LED package; the model then calculates spectral properties, CRI, LE, and LED efficiency.
Table 1. Simulated FWHM Dependence for LE at 3000 K and 90 CRI
The model predicts the maximum LE while achieving 3000 K CCT on the Planckian and CRI and R9 greater than 90 and 50, respectively. This fulfills the California utility commission standard and helps adoption of LED lighting by reproducing the color quality of incandescent lighting. The FWHM is reduced from 110 to 30 nm for green and 90 to 30 nm for red while the peak wavelength is optimized for maximum LE. The blue LED peak wavelength was fixed at 450 nm and constant FWHM of 16 nm. When the FWHM of the red phosphor is reduced, there is a dramatic increase in LE. However, the same reduction in the green phosphor realizes a smaller change. The reduction of red FWHM from 90 to 30 nm improves the LE by 13% whereas the same 60 nm reduction in the green from 110 to 50 nm improves the LE by only 4%. The 30 nm green with 30 or 50 nm red FWHM were not calculated as there are no solutions to meet the required CCT and CRI for a single red and green Gaussian emission mixture. It is also important to highlight the sensitivity of the maximum LE on the peak wavelength. For the QD based LED example, we selected the 40 nm FWHM red Gaussian peak and the 110 nm FWHM green Gaussian peak to represent the red QD and the yttrium aluminum garnet phosphor, respectively. In this combination, the LE is reduced by for each nanometer of wavelength redshifted from the optimal position. The customized peak wavelength is a subtle but significant parameter that is uniquely addressed by QDs due to their continuous tunability.
Figure 2(a) expands the QD based LED model over the range of warm (2700 K) to cool (6500 K) white CCTs at 70, 80, and 90 CRI values and compares the predicted maximum LE to measured LE performance of commercial LED phosphors. This shows that the theoretical LE gains are highest for 90 CRI due to the demanding spectral matching to the blackbody source. At 80 and 70 CRI the LE gains are reduced but still meaningful even up to 6500 K at a CRI of 70 or 80. The increasing LE gains for lower CCT values makes intuitive sense since there is a greater portion of red photons that make up the warm white light spectrum; this quantifies how the narrow red QD lead to benefits over conventional broad red phosphor.
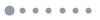
Figure 2.(a) Modeling results showcasing LE performance expectations based on a 40 nm FWHM red QD compared to red nitride based pcLED. (b) Experimentally measured QD based LED CE normalized to a commercial red nitride based pcLED at different CCT values.
B. LED Efficiency
In Fig. 2(b), we show the experimental results of QD based LUXEON 3535L HE Plus white LEDs at 90 CRI and various CCTs at a heat sink temperature of 60°C driven at 65 mA [16], typical for a downlight application. The QD based LED shows significant conversion efficiency (CE) improvement over the commercial red nitride based phosphor converted LED (pcLED). The CE is defined as the product of the package efficiency, LE, the quantum efficiency of down conversion, and the Stokes shift efficiency at the longer wavelengths. The CE gains are highest at 2700 K approaching 15% gains and still above 6% at 5000 K. In comparing the LE gains of Fig. 2(a) and the CE gains of Fig. 2(b), the CE gain is about 5% lower than the theoretical maximum LE gains calculated from the model. This difference can be attributed to a combination of QE and optical scattering differences between the QD and the red nitride phosphor and the nonoptimization of the QD peak wavelength for each CCT, CRI, and operating temperature. The operating temperature is also important because the QD peak can redshift by for a 10°C increase in temperature.
In Fig. 3(a), the CE performance of a 3000 K, 90 CRI QD based LED over a conventional pcLED is measured to higher drive currents and at 85°C heat sink temperature that would represent operating conditions for a retrofit light bulb. The comparison between the QD and the red nitride based LED shows 10%–12% gain at drive currents ranging from 30 to 400 mA. The QD based LED color shift over this range, shown in Fig. 3(b), is pts and pts in CIE 1976 color space, which is comparable to the red nitride phosphor based LED. The slightly larger color shift for the QD based LED is due to more reversible photothermal quenching of the QDs, which will be discussed in more detail later in the paper. The red QD temperature dependent peak wavelength shift and FWHM changes have minimal impact on this color point shift.
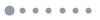
Figure 3.(a) Measured CE performance versus drive current and (b) color point shift comparison between red nitride phosphor and QD based LED at 3000 K, driven in DC mode and 85°C heatsink temperature.
Table 2 lists external testing results from LEDs in Figs. 3 and 4 that were assembled into an LED module. This example module contains an array of 15 LEDs on a printed circuit board with three strings wired in parallel. The QD and the red nitride phosphor based LEDs were matched with blue LED chips within 2.5 nm wavelength distribution so that the difference in performance can be attributed to the white light conversion only.
Sample | Current (mA) | CCT (K) | CRI | R9 | Luminous Flux (lm) | Efficacy (lm/W) |
QD based pcLED module A | 225 | 2966 | 89 | 52 | 497 | 160 |
Phosphor based pcLED module B | 225 | 3061 | 89 | 45 | 439 | 142 |
258 | 3057 | 89 | 44 | 495 | 137 |
Table 2. External Testing Results of a QD Based LED and a pcLED Module on Printed Circuit Board
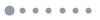
Figure 4.Reliability of QD LEDs under 95°C and 200 mA HTOL conditions. (a) Light output maintenance and (b) color shift over 3000 h duration.
Care was taken to ensure that the LED modules measured similar color points, CRI, and R9 values. When driven at the same current density, the QD based LED module produced a 13% luminous efficiency improvement compared to the red nitride based module, consistent with the results from Fig. 3. In the case where the LEDs are compared at the same lumen output, the red nitride based LEDs need to be driven at a higher drive current to match the lumen output. The QD based LEDs then provide 17% efficiency gains over the red nitride based LED. Because of the significant current density droop effect of InGaN LEDs, the changes in CE have a nonlinear impact on the total LED module. Additionally, as the total efficiency of the module improves, the LED heatsink can be reduced in cost and size.
C. Reliability
The use of QDs as a narrow red solution for white LEDs has been discussed for some time [17] but the biggest obstacle has been the fast degradation of QDs under LED operating conditions. In Figs. 4 and 5, we show the high temperature operating life (HTOL) and high humidity or wet HTOL (WHTOL) stress testing results. Figures 4(a) and 4(b) show LED HTOL stability in terms of light output power and color shifts after operating at 95°C and being driven at 200 mA for 3000 h.
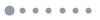
Figure 5.Reliability of QD LEDs under 60°C/90% RH WHTOL stress conditions. (a) Light output maintenance and (b) color shift over 1000 h duration.
At these conditions, the QD temperature can reach over 110°C under blue flux intensities of ; however, the comparison to the red nitride phosphor based LED shows that the QD based LED can maintain light output and color stability in a similar range and within specifications. Although the long-term testing is not complete at the time of preparing this paper, the 3000 h of testing show great promise that the color maintenance of marked in the dotted line should exceed 6000 h. Figure 5 also shows LED WHTOL stability in terms of light output power and color shifts after operating at 60°C and 90% relative humidity and driven at 65 mA for 1000 h where the dotted line marks the maximum color shift allowed under WHTOL testing. Both the WHTOL and HTOL tests show color shifts below at 1000 and 3000 h, respectively. This shows that the QDs are meeting the required lifetimes, and the prior limitations from the surface sensitivity to oxidation and defect generation have been addressed [18,19].
Typical commercially available QD materials have a core–shell QD architecture with organic ligands as surfactants passivating the surface. These QD materials can offer high quantum efficiency, narrow FWHM, and fully tunable peak wavelength emission. However, they also showed severe reversible thermal quenching and catastrophic nonreversible photothermal degradation. The significant difference in this paper is the development of a proprietary QD material where a protective barrier is designed at the QD level and the careful QD processing that has made these achievements possible. In addition, the use of QDs in a midpower LED package offers an entry point for on-chip LED applications at the moderate range of blue light intensities. For example, the blue light intensity at the surface of the LED would be . On the other hand, a flip-chip high power LED could output of blue light intensity.
D. Alternatives to QDs
The Philips L-prize bulb, introduced on Earth Day 2012, was the first commercial 60 W equivalent LED light bulb to the mass market with high color quality and high efficiency performance. This was made possible by combining a red LED and an off-white garnet based pcLED. We present a case study to look at such an example and how it compares to the QD based pcLED. To represent the now discontinued L-prize bulb with updated commercial LEDs, a red-orange, LUXEON C LED (618 nm peak wavelength and 15 nm FHWM at 25°C) was combined with a garnet phosphor filled LUXEON 3535L HE Plus, whereby the spectral mixing was applied from separate LED measurements following the procedure and simulator from Ohno [20]. The use of a direct red LED eliminates the inherent Stokes energy loss mechanism associated with phosphor conversion. However, the spatial separation of the red and white LEDs requires additional mixing in the LED module with secondary optics to produce a uniform white light for the consumer.
In Fig. 6, we plot the luminous efficacy of 16 off-white LEDs mixed with two red LEDs against 18 QD based LEDs. We calculate an optical mixing efficiency penalty of 13% for the hybrid LED system [21]. The LEDs were driven to produce lumen output at 3000 K and 90 CRI. The hybrid direct red LED system shows higher efficiency compared to the QD based LED at 25°C even after accounting for the optical mixing penalty, but as the temperature increases to 85°C, the red LED requires much higher drive current to maintain the same color point due to the thermal quenching of the AlInGaP LED. The dramatically reduced red LED performance translates into a higher overall efficiency for the QD based LED. An additional advantage of the QD based LED is the simplicity and cost savings of the electronic components for the overall light fixture as the red LEDs need a separate driver with temperature compensation in addition to the driver for the InGaN pumped off-white LEDs.
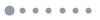
Figure 6.LED efficiency comparison between QD based LED and hybrid direct Red + pcWhite LED modules at different temperatures.
Although red QDs have shown great improvements recently in providing reliability similar to conventional phosphors, QDs still have an opportunity for further improvement. Figure 7 shows the normalized quantum efficiency comparison between (SLA) [22]—a 50 nm narrow red phosphor that emits at 650 nm—a broad read nitride phosphor, and the red QD material. Figure 7(a) shows the reversible thermal quenching behavior of the QDs compared to the SLA and a broad red nitride phosphor at , and Fig. 7(b) shows the reversible photoquenching effect at 100°C. In street lighting or automotive headlight applications, high power LEDs can reach blue light intensities in excess of , where the QDs show a larger photoquenching effect compared to the SLA phosphor. Thus, for lower temperature () and lower drive conditions (), we see the high value of QDs based on the tunable wavelength; however, at higher drive current and higher temperature, SLA would be the better solution. Other future QD improvements could include narrower FWHM and temperature independence of the emission peak wavelength.
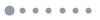
Figure 7.Normalized QE showcasing (a) thermal droop at and (b) photoquenching comparison at 100°C between QD, broad red nitride, and SLA phosphor. Inset shows their respective emission spectra.
E. Cadmium
The QDs utilized in this paper are based on II–VI semiconductors and contain cadmium. In the QD based LEDs, the Cd concentration ranges between and based on CCT; this equates to a range between and of Cd per LED. QDs can be made with alternative non-Cd materials, but the quantum efficiency and reliability have not been resolved in other systems such as Si [23], InP based [24], Pb perovskite [25], [11], and Mn-doped ZnSe QDs [26]. There are also other challenges with alternative noncadmium materials such as limitations in achieving narrow FWHM, full tunability of the emission, or insufficient absorption at 450 nm. Finally, new materials such as Pb perovskite QDs may have similar toxicity concerns.
The first production-ready on-chip LED demonstration with Cd based QD material is a true breakthrough for QD technology. QDs prepared via a colloidal route can be made to operate at high temperature, high humidity, and under high intensity light flux for very long times. This existence proof paves the way for improved stability for alternative QD materials and for QDs of various colors such as in display or horticultural LED applications.
F. Cost
The on-chip application of QDs in midpower LEDs meets the prerequisite for commercial success by managing the additional bill of materials cost of QDs. The maturity of LEDs for general illumination has seen dramatic cost reductions in the LED lamps and luminaires over the past few years. This has also resulted in more universal designs and commoditization of LEDs such that the semiconductor chip, silicone encapsulant, lead frame package, and phosphor have been optimized for low cost. The QD performance advantage has a clear upper limit cost metric, that is, the incremental cost of adding additional LEDs to provide the same efficacy and lumen output. There are some applications where the LED surface area is limited (e.g., small form factor lamps such as GU10 and MR16), and here slightly higher cost can be accepted. However, remote phosphor applications in general illumination are too expensive for widespread adoption, reaching at most 3% market share [8]. Such examples include the seminal Nexxus Lighting/QDVision lightbulb [27], which set a precedent for delivering red QDs into lighting with a remote QD component placed atop the light fixture and the aforementioned Philips L-prize bulb. As the market moves to light bulbs below the $5 price point, the phosphor and/or QD must be applied within the package.
4. CONCLUSIONS
QD based LEDs have shown promise for over a decade after the first demonstration of color conversion with a blue LED [28]. The combination of narrow FHWM and tunable peak wavelength of red QDs has resulted in 17% efficiency improvement of QD based LEDs compared to commercial red nitride based LEDs. The LED stability with of incident blue light and QD temperatures over 110°C have passed 3000 h and continue to show no degradation.
Acknowledgment
Acknowledgment. The authors would like to thank Helen Gan, Jacques Heuts, Frank Jin, Sheila Morgan, and Mark Spencer for all of their assistance, and Pacific Light Technologies for their collaboration in providing the QD materials and thoughtful technical discussions.
References
[1] A. P. Alivisatos. Semiconductor clusters, nanocrystals, and quantum dots. Science, 271, 933-937(1996).
[2] D. V. Talapin, A. L. Rogach, A. Kornowski, M. Haase, H. Weller. Highly luminescent monodisperse CdSe and CdSe/ZnS nanocrystals synthesized in a hexadecylamine-trioctylphosphine oxide-trioctylphospine mixture. Nano Lett., 1, 207-211(2001).
[3] S. F. Wuister, I. Swart, F. van Driel, S. G. Hickey, C. D. Donega. Highly luminescent water-soluble CdTe quantum dots. Nano Lett., 3, 503-507(2003).
[4] Y. Gao, X. Peng. Photogenerated excitons in plain core CdSe nanocrystals with unity radiative decay in single channel: the effects of surface and ligands. J. Am. Chem. Soc., 137, 4230-4235(2015).
[5] P. Reiss, J. Bleuse, A. Pron. Highly luminescent CdSe/ZnSe core/shell nanocrystals of low size dispersion. Nano Lett., 2, 781-784(2002).
[6] F. Pinaud, X. Michalet, L. A. Bentolila, J. M. Tsay, S. Doose, J. J. Li, G. Iyer, S. Weiss. Advances in fluorescence imaging with quantum dot bio-probes. Biomaterials, 27, 1679-1687(2006).
[7] H. E. Grecco, K. A. Lidke, R. Heintzmann, D. S. Lidke, C. Spagnuolo, O. E. Martinez, E. A. Jares-Erijman, T. M. Jovin. Ensemble and single particle photophysical properties (two-photon excitation, anisotropy, FRET, lifetime, spectral conversion) of commercial quantum dots in solution and in live cells. Microsc. Res. Tech., 65, 169-179(2004).
[8] E. Virey. From Technologies to Market Phosphors and Quantum Dots LED Downconverters for Lighting and Display Applications(2015).
[9] R. J. Nick, C. A. Breen, C. M. Denton, S. Sadasivan, J. R. Linton. Method of making components including quantum dots, methods and products. U.S. patent(2014).
[10] Q. Hong, K.-C. Lee, Z. Luo, S.-T. Wu. High-efficiency quantum dot remote phosphor film. Appl. Opt., 54, 4617-4622(2015).
[11] H. Kim, J. Y. Han, D. S. Kang, S. W. Kim, D. S. Jang, M. Suh, A. Kirakosyan, D. Y. Jeon. Characteristics of CuInS2/ZnS quantum dots and its application on LED. J. Cryst. Growth, 326, 90-93(2011).
[12] S. R. Cordero, P. J. Carson, R. A. Estabrook, G. F. Strouse, S. K. Buratto. Photo-activated luminescence of CdSe quantum dot monolayers. J. Phys. Chem. B, 104, 12137-12142(2000).
[13] S. Jun, J. Lee, E. Jang. Highly luminescent and photostable quantum dot-silica monolith and its application to light-emitting diodes. ACS Nano, 7, 1472-1477(2013).
[14] T. Erdem, S. Nizamoglu, X. W. Sun, H. V. Demir. A photometric investigation of ultra-efficient LEDs with high color rendering index and high luminous efficacy employing nanocrystal quantum dot luminophores. Opt. Express, 18, 340-347(2010).
[15] . LUXEON 3535L Line(2016).
[16] A. R. Duggal, A. M. Srivastava, L. M. Levinson, S. J. Duclos. Color tunable light source. U.S. patent(2002).
[17] H. Chen, C. Hsu, H. Hong. InGaN–CdSe–ZnSe quantum dots white LEDs. IEEE Photon. Technol. Lett., 18, 2005-2007(2006).
[18] J. Cui, A. P. Beyler, T. S. Bischof, M. W. B. Wilson, M. G. Bawendi. Deconstructing the photon stream from single nanocrystals: from binning to correlation. Chem. Soc. Rev., 43, 1287-1310(2014).
[19] C. Pu, X. Peng. To battle surface traps on CdSe/CdS core/shell nanocrystals: shell isolation versus surface treatment. J. Am. Chem. Soc., 138, 8134-8142(2016).
[20] Y. Ohno. Spectral design considerations for white LED color rendering. Opt. Eng., 44, 111302(2005).
[21] J. Brodrick, N. Bardsley, M. Hansen, L. Pattison, M. Pattison, K. Stober, V. Taylor, J. Tsao, M. Yamada. Solid-State Lighting 2016 R&D Plan(2016).
[22] P. Pust, V. Weiler, C. Hecht, A. Tücks, A. S. Wochnik, A. Henß, D. Wiechert, C. Scheu, P. J. Schmidt, W. Schnick. Narrow-band red-emitting Sr[LiAl3 N4]:Eu2+ as a next-generation LED-phosphor material. Nat. Mater., 13, 891-896(2014).
[23] C. Tu, J. H. Hoo, K. F. Böhringer, L. Y. Lin, G. Cao. Red-emitting silicon quantum dot phosphors in warm white LEDs with excellent color rendering. Opt. Express, 22, A276-A281(2014).
[24] S. Kim, K. Taehoon, S.-W. K. Kim. Highly luminescent InP/GaP/ZnS nanocrystals and their application to white LED SI, 1-9(2011).
[25] A. Swarnkar, R. Chulliyil, V. K. Ravi, M. Irfanullah, A. Chowdhury, A. Nag. Colloidal CsPbBr3 perovskite nanocrystals: luminescence beyond traditional quantum dots. Angew. Chem., 54, 15424-15428(2015).
[26] N. Pradhan, X. Peng. Efficient and color-tunable Mn-doped ZnSe nanocrystal emitters: control of optical performance via greener synthetic chemistry. J. Am. Chem. Soc., 129, 3339-3347(2007).
[27] A. Mnoyan, Y. Lee, H. Jung, S. Kim. Phosphors, Up Conversion Nano Particles, Quantum Dots and their Applications, 2, 437-471(2016).
[28] J. Lee, V. C. Sundar, J. R. Heine, M. G. Bawendi, K. F. Jensen. Full color emission from II ± VI semiconductor. Adv. Mater., 12, 1102-1105(2000).