Abstract
We report on a compact passive mode-locked Er:fiber ring laser operated at the fundamental repetition rate of 517 MHz, which we believe is the highest fundamental repetition rate ever reported in a ring cavity fiber laser. The key technique is the employment of two innovative high-power wavelength domain multiplexer collimators with all gain fiber cavity suited for the high power (up to 2 W) pumping. The laser is featured with a direct chirpfree output pulse, which is 97 fs without extracavity compression at an average output power of 90 mW.1. INTRODUCTION
Compact high-repetition-rate femtosecond fiber lasers have a lot of applications such as optical frequency metrology [1], arbitrary optical waveform generation [2], high-speed optical sampling, and frequency distribution via optical fiber or open atmospheric links [3]. In the application of frequency comb metrology [4–6], larger frequency spacing is favored for higher teeth intensity and for the reduced accuracy of the wavelength meter.
Fiber lasers employing a linear cavity and saturable absorber have reached the GHz level [7]. However, the pulse-shaping mechanism limits the spectral bandwidth and leads to a broader pulse. A ring cavity with mode locking started with an intracavity modulator [8] or harmonic mode locking [9] can only deliver picosecond pulses, though the repetition rate can be as high as several GHz. The importance of the pulse width is the spectral coherence generated in a high nonlinear fiber that requires seeding pulses [10]. The low pulse energy is also difficult to use for expanding the spectrum to octave spanning.
A ring cavity adapting nonlinear polarization evolution (NPE) relies on a fast saturation absorption mechanism and takes advantage of very short pulse generation [11]. Unlike the Yb fiber laser, which has a high gain, and the short fiber, which can be employed for 1 GHz repetition rate operation [12], silica-based Er-doped fiber has relatively low gain. A ring cavity with silica Er:fiber lasers with repetition rates up to 300 MHz has been demonstrated by several groups. An MIT group reported a 301 MHz Er:fiber ring-cavity laser, which is pumped by a spatially coupled 1.2 W diode [13]. Meanwhile, our group has already demonstrated a 464 MHz soliton Er:fiber laser, and the measured pulse width was as short as 123 fs without extracavity compression [14]. On the other hand, the phosphate fiber can have higher doping. By employing a 5.7 cm long high-gain co-doped phosphate glass fiber, Wei et al. demonstrated a 325 MHz all fiber ring-cavity Er:fiber laser with 105 fs pulse width but with very low output power [15]. Although highly doped phosphate fiber gives enough gain in centimeter length fiber, it is difficult to splice with the single-mode silica fiber; therefore, it has to be connected inside ferrules by optical contact, which results in a large loss.
Sign up for Photonics Research TOC. Get the latest issue of Photonics Research delivered right to you!Sign up now
Therefore, we have been trying to increase the pulse repetition rate to take advantage of high output power and short pulses.
To further increase the pulse repetition rate with silica-based Er:fiber, besides using a short fiber cavity, we have to increase the pump power. This requires removing all nongain fibers. We developed a so-called active wavelength domain multiplexer (WDM) collimator that uses implemented gain fiber as the pigtail to reduce the cavity length without losing gain. However, the fiber can be easily damaged at the pump power above 0.8 W. The solution is to enlarge the mode field size of the fiber tip inside the WDM collimator.
In this paper, we report a 517 MHz repetition rate, NPE mode-locked Er:fiber soliton fiber laser. With the anomalous dispersion gain fiber, the laser can be easily mode locked in the soliton regime.
2. DESIGN AND CONSTRUCTION OF THE FIBER LASER
To make the laser mode locked easier at such a high repetition rate, we intend to operate the laser in the soliton regime. The schematic of the cavity is illuminated in Fig. 1. We choose a 360 mm long Er80-8/125 gain fiber as the pigtail fiber of both WDM collimators, which is anomalously dispersed in the laser wavelength. The WDM collimators reflect the pump to fiber and transmit the oscillation wavelength. The free space includes a pair of gain fiber pigtailed WDM collimators, two quarter-wave plates, a 2 mm thick pill-sized isolator, a 5 mm cube polarization beam splitter, and a half-wave plate. To save the space, the wave plates are bared without mounting holders. The center of each wave plate is glued on a rotation axis. This design makes the free-space region shrink to 28 mm. The net cavity dispersion is at the central wavelength of 1560 nm.
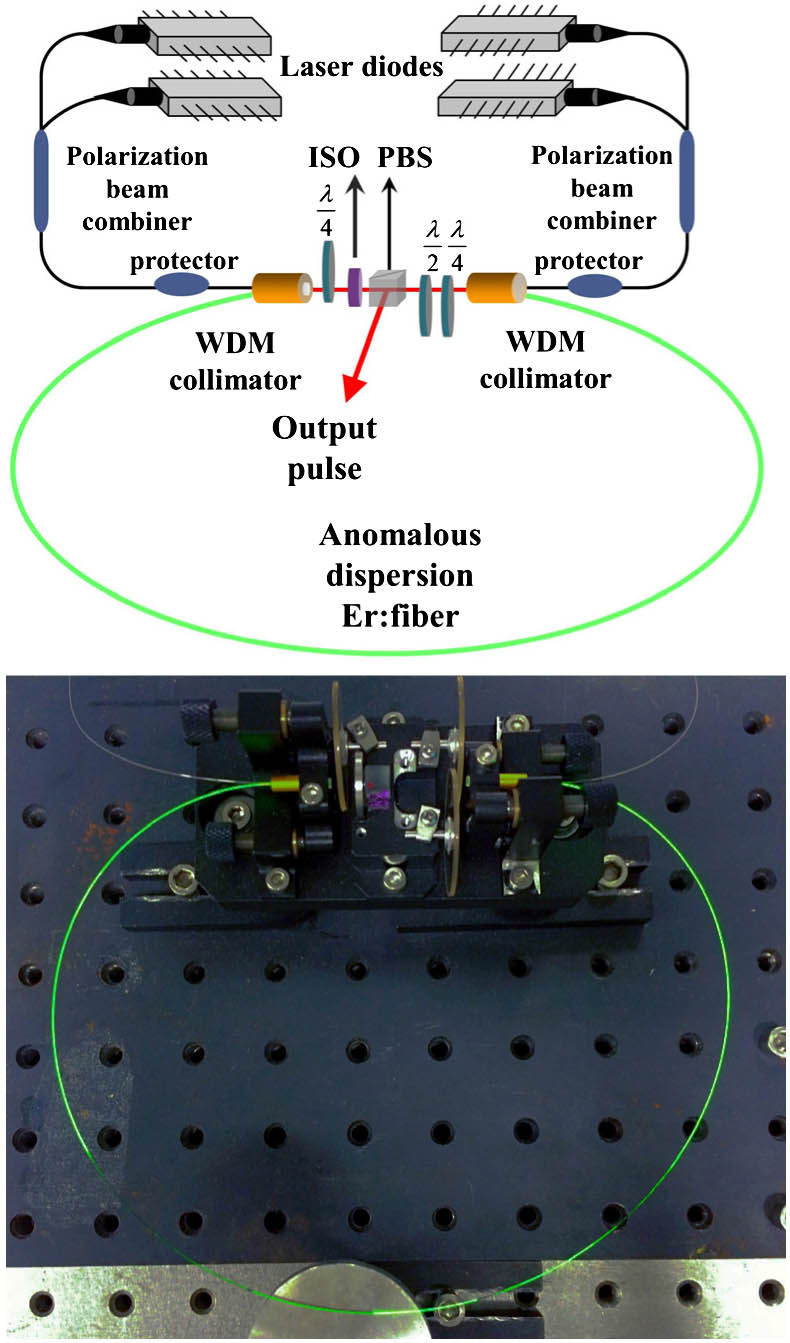
Figure 1.Schematic of the Er fiber laser. ISO, Faraday isolator; PBS, polarization beam splitter; , quarter-wave plate; , half-wave plate.
3. CHARACTERIZATION OF THE FIBER LASER
The pump power was coupled into the cavity via both WDM collimators. Because of the large difference between the pump and the laser wavelengths, the WDM has a higher loss than the 980/1030 loss for Yb:fiber lasers. The pump source is two pairs of 974 nm laser diode modules, which offer a pump power up to 1100 and 900 mW from each side, respectively. At the maximum pump power, the CW output power was 236 mW, showing an overall efficiency of 11.8%. The mode locking can be easily initialized with the standard NPE at above the pump power of 2 W. When mode-locked, the laser average output power is 90 mW. The fundamental repetition rate of the mode locking is 517 MHz (Fig. 2). The mode locking is self-starting and can sustain for a few days though exposure to the open-air environment.
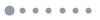
Figure 2.Radio frequency spectrum up to 3 GHz with the resolution bandwidth of 30 kHz.
The pulse spectrum is shown in Fig. 3, which is reckoned as a typical soliton. The spectrum width (FWHM) is 30 nm. The superimposed is the simulation of output spectrum, which agree well for the most part. The measured fringe-resolved autocorrelation trace of the direct output pulse (without dispersion compensation) is shown in Fig. 4. It fits fairly well with 8:1 with little wings. The pulse width is deconvoluted to 97 fs with a secant hyperbolic pulse profile and a flat phase assumed. The transform limited pulse of 82 fs is calculated from the measured spectrum, while the simulated output pulse profile is also shown in Fig. 4. There is still a small chirp in the output pulse, which needs to be corrected if necessary.
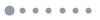
Figure 3.(a) Soliton pulse spectrum measured in linear scale (black) and simulation result (red). (b) Spectrum measured in log scale (black) and simulation result (red).
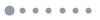
Figure 4.(a) Fringe-resolved autocorrelation trace of the direct output pulses. (b) Inset is the calculated intensity autocorrelation trace of transform-limited pulse is about 73 fs (blue line) and simulated pulse profile with 82 fs (red dot). In both cases, the pulse profiles are assumed to be secant hyperbolic.
This short pulse will benefit the subsequent power amplification and spectrum expansion for making it an optical frequency comb. However, the direct output power drops from 230 mW at CW to 90 mW at mode lock, which is not yet understood.
Figure 5 shows the simulated intracavity pulse evolution. The spectrum width envolves slightly from 31 to 33 nm in the whole cavity, which fits the property of soliton pulses. The pulse width is stretched by amplification in a previous section of gain fiber and then compressed by anomalous dispersion and self-phase modulation in the latter section. Compared with the long fiber cavities, the short fiber in the cavity offers low dispersion and leads to narrow intracavity pulse width, which ensures the interplay between the dispersion and self-phase modulation in the cavity, so as to maintain the laser mode locked. The direct output pulse is shortened to compared with our previously reported 123 fs in a 464 MHz fiber laser, which proved the benefit of the short fiber cavity. However, the output power is lower because of the shorter gain fiber. The pulse width should be shorter if dispersion compensation is introduced. However, that will ask for a higher fiber gain; otherwise, the laser is difficult to mode lock.
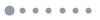
Figure 5.Evolution of pulse duration inside the laser cavity. Gain fiber, CorActive Er-doped gain fiber (Er80-8/125); NPE, saturable absorber.
4. CONCLUSION
We have demonstrated a femtosecond mode-locked Er:fiber ring laser operated at 517 MHz fundamental repetition rate. To the best of our knowledge, this is the highest fundamental repetition rate yet reported in a ring cavity fiber laser. The innovative 980/1550 WDM collimator ensures the laser remains compact and stable. The optical spectrum width is 30 nm, and the direct output pulse duration is 97 fs without extracavity compression. The output power at the pump power of 2 W is 90 mW corresponding wih the pulse energy of 0.18 nJ. Compared with our previous report on 464 MHz Er:fiber lasers [14], we can see a tendency that the direct output pulse is getting shorter at a higher repetition rate.
Further increasing the pulse repetition rate involves a higher pump power and highly doped fiber such as the phosphate glass fiber.
Acknowledgment
Acknowledgment. This work was partially supported by the Major National Basic Research Program of China (2013CB922401) and the National Natural Science Foundation of China (60927010, 10974006, and 11027404).
References
[1] J. Ye, H. Schnatz, L. W. Hollberg. Optical frequency combs: from frequency metrology to optical phase control. IEEE J. Sel. Top. Quantum Electron., 9, 1041-1058(1999).
[2] K. Jung, J. Shin, J. Kim. Ultralow phase noise microwave generation from mode-locked Er-fiber lasers with subfemtosecond integrated timing jitter. IEEE Photon. J., 5, 5500906(2013).
[3] R. P. Gollapalli, L. Duan. Multiheterodyne characterization of excess phase noise in atomospheric transfer of a femtosecond-laser frequency comb. IEEE J. Lightwave Technol., 29, 3401-3407(2011).
[4] G. Ycas, S. Osterman, S. A. Diddams. Generation of a 660–2100 nm laser frequency comb based on an erbium fiber laser. Opt. Lett., 37, 2199-2201(2012).
[5] T. Shioda, T. Yamazaki. Ultrafast optical frequency comb synthesizer and analyzer. Opt. Lett., 37, 3642-3644(2012).
[6] A. Schliesser, N. Picqué, T. W. Hänsch. Mid-infrared frequency combs. Nat. Photonics, 6, 440-449(2012).
[7] Y. Song, C. Goh, Y. Set, S. Yamashita. Passively mode-locked lasers with 17.2-GHz fundamental-mode repetition rate pulse by carbon nanotubes. Opt. Lett., 32, 430-432(2007).
[8] S. Zhang, J. Yao, Y. Liu, F. Lu. Fiber-format pico-second source based on efficient frequency doubling of a high-repetition rate erbium-fiber laser amplifier. Microwave Opt. Technol. Lett., 53, 169-171(2011).
[9] D. Mao, X. Liu, Z. Sun, H. Lu, D. Han, G. Wang, F. Wang. Flexible high-repetition-rate ultrafast fiber laser. Sci. Rep., 3, 3223(2013).
[10] P. Li, G. Wang, C. Li, A. Wang, Z. Zhang, F. Meng, S. Cao, Z. Fang. Characterization of the carrier envelope offset frequency from a 490 MHz Yb-fiber-ring laser. Opt. Express, 20, 16017-16022(2012).
[11] D. Ma, Y. Cai, C. Zhou, L. Chen, Z. Zhang. 37.4 fs pulse generation in an Er:fiber laser at a 225 MHz repetition rate. Opt. Lett., 35, 2858-2860(2010).
[12] C. Li, X. Gao, G. Wang, T. Jiang, A. Wang, Z. Zhang. 1 GHz repetition rate ring cavity femtosecond Yb:fiber laser. Conference on Lasers and Electro-Optics (CLEO), SW3E.2(2014).
[13] J. L. Morse, J. W. Sickler, J. Chen, F. X. Kärtner, E. P. Ippen. High repetition rate, high average power, femtosecond erbium fiber ring laser. Conference on Lasers and Electro-Optics (CLEO), CML1(2009).
[14] J. Zhang, F. Niu, X. Chen, X. Gao, Y. Liu, A. Wang, Z. Zhang. 464 MHz repetition rate erbium doped soliton fiber laser. Conference on Lasers and Electro-Optics (CLEO), SW3E.1(2014).
[15] X. Wei, S. Xu, H. Huang, M. Peng, Z. Yang. Compact all-fiber ring femtosecond laser with high fundamental repetition rate. Opt. Express, 20, 24607-24613(2012).