Author Affiliations
1School of Electronic and Information Engineering, Beihang University, Beijing 100083, China2Yunnan Key Laboratory of Opto-Electronic Information Technology, School of Physics and Electronic Information Technology, Yunnan Normal University, Kunming 650500, China3Beijing Advanced Innovation Center for Big Data-Based Precision Medicine, Beihang University, Beijing 100083, China4Collaborative Innovation Center of Geospatial Technology, Wuhan 430079, Chinashow less
Abstract
Mode-locked fiber lasers that can simultaneously generate two asynchronous ultrashort pulse trains could play an attractive role as the alternative light sources for low-complexity dual-comb metrology applications. Although a few multiplexing schemes to realize such lasers have been proposed and demonstrated, here we investigate the lasing characteristics of a passively mode-locked fiber laser with a finite amount of intracavity birefringence. By introducing a section of polarization-maintaining (PM) fiber into the otherwise-non-PM-single-mode cavity, dual asynchronous pulses with nearly orthogonal states of polarization are generated. With a repetition rate difference of hundreds of hertz, the pulses have well-overlapped spectra and show typical features of polarization-locked vector solitons. It is demonstrated that under an anomalous or net normal dispersion regime, either dual vector solitons or dual dissipative vector solitons can be generated, respectively. Such polarization-multiplexed single single-cavity dual-comb lasers could find further uses in various applications in need of simple dual-comb system solutions.1. INTRODUCTION
Two optical frequency combs with slightly different comb-tooth spacing can enable a number of dual-comb metrology applications [1], such as high-resolution spectroscopy and ranging. Because the traditional implementations based on two frequency-stabilized ultrafast lasers [2] are considered by many as overly complicated or technically challenging, the efforts to simplify such systems have intensified in the past few years. Using two free-running mode-locked lasers, digital phase correction algorithms or adaptive-sampling data acquisition systems can compensate their repetition rate drifts [3,4]. It was also found that sharing one common optical parametric oscillator cavity for wavelength-conversion of the pulses from two free-running seed lasers could improve the dual-comb spectroscopy measurement performance [5] by reducing the random drifts in two cavities. The outputs from two mode-locked waveguide lasers [6] or nonlinear microresonators [7] fabricated and operated on the same chip had been shown to possess sufficient mutual coherence for certain dual-comb applications with large comb-tooth spacing. Two electro-optic combs seeded by one continuous-wave (CW) laser followed by nonlinear spectral broadening had been applied to spectroscopic measurement by setting slightly different modulation frequencies [8]. While having flexible choices of the center wavelength and comb-tooth spacing, a compromise between their comb-tooth spacing and spectral bandwidth could exist.
Generating dual-comb pulses directly from one seed cavity instead of two, however, seems to be an attractive and promising approach because of the possible higher degree of mutual coherence between the pulses. There had been increasingly strong interest in realizing such a single-cavity dual-comb source (SCDCS) on various laser platforms. Several schemes of generating asynchronous ultrashort pulses from a mode-locked fiber laser have been demonstrated by leveraging dual-wavelength [9], bidirectional [10–12] lasing, or nonlinear pulse-shaping mechanisms [13]. Dual-comb pulse generation had also been realized in a bidirectionally oscillating Ti:sapphire laser [14] as well as a bidirectionally pumped microresonator [15]. Orthogonally polarized dual-comb generation was also achieved in a semiconductor disk laser by spatially separating the beam paths in the cavity with a birefringent crystal [16]. Such SCDCSs with asynchronous pulse emissions had demonstrated their potential in several dual-comb applications as simple, alternative dual-comb sources [17–21]. Among them, fiber lasers with low complexity and high energy efficiency have relatively small comb-tooth spacing and potentially large spectral coverage.
Besides the aforementioned dual-comb fiber lasing schemes, the laser source with polarization-multiplexed outputs could generate dual pulses with orthogonal polarization states in the same spectral window [22,23]. In contrast to the so-far mostly investigated dual-wavelength SCDCS for dual-comb applications, its output can be spectrally coherent and have no inherent bandwidth limitation. Whereas polarization-maintaining (PM) fiber lasers emit light at one polarization, there have been numerous studies on the state of polarization of pulses generated by traditional mode-locked fiber lasers consisting of standard single-mode fibers (SMFs). It has been shown that vector soliton or dissipative vector soliton can be generated under anomalous or normal net cavity dispersion, respectively, in such a fiber laser with weak intracavity birefringence (mostly introduced by the residual fiber/component birefringence in the cavity) [24–27]. Synchronous oscillation of more than one vector solitons with orthogonal state of polarization had been also observed, likely due to the spatial gain hole burning effect in the laser [26].
Sign up for Photonics Research TOC. Get the latest issue of Photonics Research delivered right to you!Sign up now
In this paper, we demonstrate a simple yet effective scheme of polarization-multiplexed, asynchronous pulse generation from a fiber laser. It is demonstrated that both vector solitons and dissipative vector solitons with a small repetition rate difference can be obtained from the laser under either negative or positive net cavity group velocity dispersion (GVD).
2. EXPERIMENTAL SETUP
In contrast to the traditional SMF laser or the PM one, our laser configuration is based on a partially birefringent design. The setup of the dual-comb mode-locked laser shown in Fig. 1 consists of an all-fiber ring configuration where the pulses would propagate over a completely common-path route in the cavity instead of a partially shared one. To enable dual-comb lasing, a section of PM fiber (PMF) (0.30 m long in this case, with a beat length of ) is placed in the ring, which introduces some limited but considerable birefringence that can result in significant group velocity difference for pulses with different states of polarization. It plays a critical role to enable forming multiple asynchronous vector solitons simultaneously. The rest of the ring consists of a 0.38 m long piece of erbium-doped fiber (EDF, Fibercore Er110) forward pumped by a 980 nm pump diode through a wavelength division multiplexer (WDM) with a 0.31 m long Hi1060 pigtail in the laser cavity. A polarization-independent isolator (ISO) and a polarization controller (PC) are used to ensure the unidirectional operation and control the polarization evolution, respectively. A single-wall carbon nanotube saturable absorber (SWNT-SA) fabricated by the optical deposition method is used to enable mode locking. The dual combs are coupled out of the laser by an optical coupler (OC). For polarization-resolved measurements of the laser output, a polarization beam splitter (PBS) following a PC is used to split the light into two arms, denoted as Pol-1 and Pol-2. A PC is used to adjust the alignment of the output’s state of polarization to the axis of the PBS.
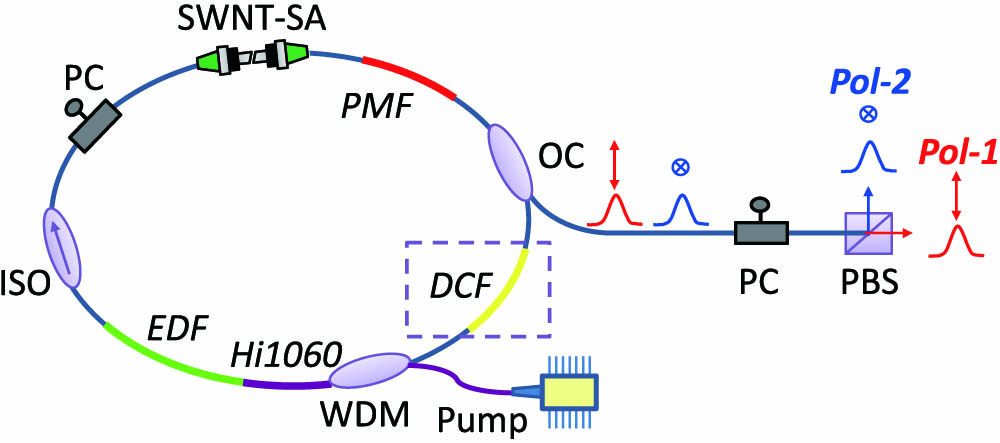
Figure 1.Schematic of the polarization-multiplexed, dual-comb fiber laser.
To investigate dual-comb generation under different dispersion regimes, the net dispersion can be adjusted. When the total length of SMF in the cavity is , the net cavity GVD is estimated to be . Here, the GVD parameters of EDF, Hi1060, PMF, and SMF at 1550 nm are estimated as 12.2, , , and , respectively. The net negative GVD can lead to soliton operation. By adding a section of 2.09 m long dispersion compensating fiber (DCF) (GVD ) with a 0.24 m long SMF pigtail, the net cavity GVD can be shifted to that could enable dissipative soliton generation.
3. EXPERIMENTAL RESULTS
First, when the laser is operated with the anomalous net GVD, the laser starts self-mode locking at pump power. After the pump power is increased to , by properly adjusting the intracavity polarization state, an additional pulse train can be observed on an oscilloscope (Agilent MSO 7054A) following the 1 GHz bandwidth fast photodetector. Figure 2(a) shows an oscilloscope screenshot when the laser output is measured by the photodetector directly. Within the 100 μs time window, the temporal separation between pairs of pulses in the zoom-in waveforms at each end changes by more than 1 ns. This indicates that the pulses have slightly different repetition rates, which is further validated by later measurements.
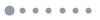
Figure 2.(a) Oscilloscope trace, (b) optical spectra, (c) RF spectra, (d) oscilloscope traces of two polarizations, and (e) AC traces, of the dual pulses when the laser operates at the DVS state.
The optical spectrum of the laser output now still has a soliton shape with two side peaks often seen in vector solitons, as shown in Fig. 2(b). When measured with the photodetector and a radio frequency (RF) spectrum analyzer (Agilent E4404B), two frequency components at 44.102951 and 44.103377 MHz, respectively, can be observed around 44.1 MHz, which corresponds to the cavity length, as seen in Fig. 2(c). This further demonstrates that, in contrast to the synchronous pulses observed in Ref. [26], two generated pulse trains have stable but different repetition rates. A 426 Hz repetition rate difference can be observed between them. We note that such asynchronous pulse lasing had not been observed when the PMF is absent in the cavity. To further characterize the laser output, it is separated by the external PBS into two. By adjusting the PC before the PBS to maximize the RF signal at the lower repetition frequency for Pol-1, it can be seen that the higher frequency signal is correspondingly suppressed by more than 20 dB but not eliminated. Under this condition, the RF signal observed at Pol-2 port has the other frequency maximized, as in Fig. 2(c). This indicates that two pulse trains could have close-to-orthogonal states of polarization, although neither is linearly polarized. The temporal shapes of the pulse trains obtained from the oscilloscope are shown in Fig. 2(d). They have uniform amplitudes with negligible pulse-to-pulse modulation [26]. This suggests that both pulses could be polarization-locked vector solitons.
The optical spectra measured at Pol-1 and Pol-2 are also shown in Fig. 2(b). It can be observed that their optical spectra have quite similar shapes, except some small spectral features. The center wavelengths of Pol-1 and Pol-2 are around 1558.9 and 1558.7 nm, which are offset by only . Their 3 dB spectral bandwidths are similar as well, 3.2 and 3.4 nm, respectively, and their output powers are 635 and 490 μW. The presence of small peaks/dips pairs in the spectra, such as the one around 1570 nm with different shapes, is a typical feature for vector solitons, due to coherent energy exchange between different field components. The autocorrelation (AC) traces of the dual pulses are also measured by a home-built autocorrelator and shown in Fig. 2(e). Their 3 dB pulse width is and 713 fs, respectively, if a pulse shape is assumed. Considering the spectral bandwidth, it is believed that the laser is operated in a dual vector soliton (DVS) state generating two asynchronous vector soliton sequences with nearly orthogonal polarizations.
Furthermore, it is observed that the repetition rate difference can be varied by tuning the intracavity PC. Figure 3 shows the RF and optical spectra when is tuned from to 773 Hz. As the center wavelength separation of the pulses only slightly varies, the tuning of is believed to be mainly due to the change in the composite birefringence in the cavity considering the existence of birefringence introduced by components like the ISO. This can also be seen as the position of two side peaks on the optical spectra shifts accordingly. It is noted that when the PC is adjusted to further lower , dual-comb lasing will stop with only one pulse sequence left to oscillate.
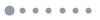
Figure 3.(a) RF spectra and (b) optical spectra when the repetition rate difference is varied.
The ability to maintain good stability in the repetition rate difference is critical for many potential dual-comb applications [14,20]. Figure 4 shows the monitored repetition rates and their difference. is set at in this case. Whereas the pulse repetition rates from the free-running laser drift noticeably as expected, remains steady with a root-mean-square of only 38 mHz.
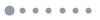
Figure 4.Monitored repetition rates and their difference of the laser output.
When the net dispersion of the laser is changed to normal by the application of DCF as described in Section 2, two asynchronous pulse trains can also be generated, when the pump power is set above . The spectra of the dual-comb output shown in Fig. 5(a) now have a shape typical to dissipative solitons. Their bandwidths of Pol-1 and Pol-2 are 5 and 4.1 nm, respectively. They still show similar polarization and temporal characteristics based on Figs. 5(b) and 5(c). The repetition rates are 29.445086 and 29.445341 MHz with a of 255 Hz, respectively, due to the increased cavity length. Like many other dissipative soliton lasers, it is not possible to directly measure their AC at the laser output. After transmitting through 130 and 180 m SMF, respectively, 1 and 1.3 ps wide AC traces (assuming shape) are obtained after compression [see Fig. 5(d)]. This shows the strong chirp of the pulses from the laser. Therefore, it is believed that polarization-multiplexed, dual dissipative vector soliton (DDVS) generation is realized from this net normal dispersion fiber laser setup.
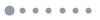
Figure 5.(a) Optical spectra, (b) RF spectra, (c) oscilloscope traces, and (d) AC traces (after compression) of the pulses at the DDVS state.
4. CONCLUSIONS
We propose and demonstrate that polarization-multiplexed SCDCS can be realized by a mode-locked fiber laser with non-negligible birefringence. A relatively small repetition rate difference around a few hundreds of hertz could be observed, which can be dynamically tuned. As it is shown that under different dispersion regimes, dual-comb pulses sharing the same spectral window could be generated with different optical bandwidths and pulse widths, it could be possible to further explore direct generation of dual-comb pulses with further increased bandwidths through intracavity dispersion design. Due to its similarity to the widely studied fiber ring lasers, this simple and easy-to-implement laser configuration could be further applied to dual-comb applications.
References
[1] I. Coddington, N. Newbury, W. Swann. Dual-comb spectroscopy. Optica, 3, 414-426(2016).
[2] L. C. Sinclair, I. Coddington, W. C. Swann, G. B. Rieker, A. Hati, K. Iwakuni, N. R. Newbury. Operation of an optically coherent frequency comb outside the metrology lab. Opt. Express, 22, 6996-7006(2014).
[3] J. Roy, J.-D. Deschênes, S. Potvin, J. Genest. Continuous real-time correction and averaging for frequency comb interferometry. Opt. Express, 20, 21932-21939(2012).
[4] T. Ideguchi, A. Poisson, G. Guelachvili, N. Picqué, T. W. Hänsch. Adaptive real-time dual-comb spectroscopy. Nat. Commun., 5, 3375(2014).
[5] Y. Jin, S. M. Cristescu, F. J. Harren, J. Mandon. Two-crystal mid-infrared optical parametric oscillator for absorption and dispersion dual-comb spectroscopy. Opt. Lett., 39, 3270-3273(2014).
[6] N. B. Hébert, J. Genest, J.-D. Deschênes, H. Bergeron, G. Y. Chen, C. Khurmi, D. G. Lancaster. Self-corrected chip-based dual-comb spectrometer. Opt. Express, 25, 8168-8179(2017).
[7] A. Dutt, C. Joshi, X. Ji, J. Cardenas, Y. Okawachi, K. Luke, A. L. Gaeta, M. Lipson. On-chip dual-comb source for spectroscopy. Sci. Adv., 4, e1701858(2018).
[8] G. Millot, S. Pitois, M. Yan, T. Hovhannisyan, A. Bendahmane, T. W. Hänsch, N. Picqué. Frequency-agile dual-comb spectroscopy. Nat. Photonics, 10, 27-30(2016).
[9] X. Zhao, Z. Zheng, L. Liu, Y. Liu, Y. Jiang, X. Yang, J. Zhu. Switchable, dual-wavelength passively mode-locked ultrafast fiber laser based on a single-wall carbon nanotube modelocker and intracavity loss tuning. Opt. Express, 19, 1168-1173(2011).
[10] S. Mehravar, R. A. Norwood, N. Peyghambarian, K. Kieu. Real-time dual-comb spectroscopy with a free-running bidirectionally mode-locked fiber laser. Appl. Phys. Lett., 108, 231104(2016).
[11] X. Zhao, Z. Zheng, Y. Liu, G. Hu, J. Liu. Dual-wavelength, bidirectional single-wall carbon nanotube mode-locked fiber laser. IEEE Photon. Technol. Lett., 26, 1722-1725(2014).
[12] J. Hongbo, Y. Wang, S. Y. Set, S. Yamashita. Bidirectional mode-locked soliton fiber laser in 2 μm using CNT saturable absorber. Laser Congress (ASSL, LAC), JM5A.21(2017).
[13] Y. Liu, X. Zhao, G. Hu, C. Li, B. Zhao, Z. Zheng. Unidirectional, dual-comb lasing under multiple pulse formation mechanisms in a passively mode-locked fiber ring laser. Opt. Express, 24, 21392-21398(2016).
[14] T. Ideguchi, T. Nakamura, Y. Kobayashi, K. Goda. Kerr-lens mode-locked bidirectional dual-comb ring laser for broadband dual-comb spectroscopy. Optica, 3, 748-753(2016).
[15] Q.-F. Yang, X. Yi, K. Y. Yang, K. Vahala. Counter-propagating solitons in microresonators. Nat. Photonics, 11, 560-564(2017).
[16] S. Link, D. Maas, D. Waldburger, U. Keller. Dual-comb spectroscopy of water vapor with a free-running semiconductor disk laser. Science, 356, 1164-1168(2017).
[17] X. Zhao, Z. Zheng, L. Liu, Q. Wang, H. Chen, J. Liu. Fast, long-scan-range pump-probe measurement based on asynchronous sampling using a dual-wavelength mode-locked fiber laser. Opt. Express, 20, 25584-25589(2012).
[18] B. Lin, X. Zhao, M. He, Y. Pan, J. Chen, S. Cao, Y. Lin, Q. Wang, Z. Zheng, Z. Fang. Dual-comb absolute distance measurement based on a dual-wavelength passively mode-locked laser. IEEE Photon. J., 9, 7106508(2017).
[19] M.-G. Suh, K. J. Vahala. Soliton microcomb range measurement. Science, 359, 884-887(2018).
[20] X. Zhao, G. Hu, B. Zhao, C. Li, Y. Pan, Y. Liu, T. Yasui, Z. Zheng. Picometer-resolution dual-comb spectroscopy with a free-running fiber laser. Opt. Express, 24, 21833-21845(2016).
[21] G. Hu, T. Mizuguchi, X. Zhao, T. Minamikawa, T. Mizuno, Y. Yang, C. Li, M. Bai, Z. Zheng, T. Yasui. Measurement of absolute frequency of continuous-wave terahertz radiation in real time using a free-running, dual-wavelength mode-locked, erbium-doped fibre laser. Sci. Rep., 7, 42082(2017).
[22] Z. Gong, X. Zhao, G. Hu, J. Liu, Z. Zheng. Polarization multiplexed, dual-frequency ultrashort pulse generation by a birefringent mode-locked fiber laser. Conference on Lasers and Electro-Optics (CLEO), JTh2A.20(2014).
[23] A. E. Akosman, M. Y. Sander. Dual comb generation from a mode-locked fiber laser with orthogonally polarized interlaced pulses. Opt. Express, 25, 18592-18602(2017).
[24] S. T. Cundiff, B. C. Collings, N. N. Akhmediev, J. M. Soto-Crespo, K. Bergman, W. H. Knox. Observation of polarization-locked vector solitons in an optical fiber. Phys. Rev. Lett., 82, 3988-3991(1999).
[25] B. C. Collings, S. T. Cundiff, N. N. Akhmediev, J. M. Soto-Crespo, K. Bergman, W. H. Knox. Polarization-locked temporal vector solitons in a fiber laser: experiment. J. Opt. Soc. Am. B, 17, 354-365(2000).
[26] L. M. Zhao, D. Y. Tang, H. Zhang, X. Wu. Polarization rotation locking of vector solitons in a fiber ring laser. Opt. Express, 16, 10053-10058(2008).
[27] H. Zhang, D. Y. Tang, L. M. Zhao, X. Wu, H. Y. Tam. Dissipative vector solitons in a dispersion-managed cavity fiber laser with net positive cavity dispersion. Opt. Express, 17, 455-460(2009).