Abstract
We propose and demonstrate a passively harmonic mode-locked erbium-doped fiber laser (EDFL) using carbon nanotubes polyvinyl alcohol (CNTs-PVA) film. The laser allows generation of the pulses with a repetition rate of 580 MHz, which corresponds to the 22nd harmonics of a 26.3 MHz fundamental repetition rate under 323 mW pump power. A particularly noteworthy feature of the pulses is the super-mode suppression ratio (SMSR), which is over 40 dB, indicating a stable operation.In recent years, a high repetition rate fiber laser was intensively explored, which is able to pave a promising way for the applications of optical frequency metrology[1], optical communication[2], as well as high speed optical sampling[3]. Ordinarily, the methods for realizing high repetition pulses focus on three aspects. An impactful approach is actively mode-locking, which is in a position to scale up the repetition rate to a few tens of gigahertz easily, while extra modulators incorporated in a laser will add a level of complexity[4,5]. Additionally, an alternative mean is to employ a short cavity. Unfortunately, the cavity length, to a great extent, is restrained by the physical size of fiber devices and limited pump power[6–8]. Furthermore, harmonic mode-locking (HML) can be adopted to sidestep the drawback of the limitation of fiber length and achieve high repetition rate simultaneously, where multiple solitons due to high pump power[9,10] organize themselves and exhibit equal spacing between adjacent pulses under a specific condition[11,12].
Thus far, a passive mode-locking (PML) fiber laser with some unique features of compact size, superior heat dissipation performance, and fairly easy maintenance has motivated much interest. There are various approaches to obtain mode-locked pulses, such as nonlinear polarization rotation (NPR)[13,14], nonlinear optical loop mirror (NOLM)[15], semiconductor saturable absorber mirror (SESEAM)[16], and the carbon-based saturable absorbers (SAs), including nanoscale graphite[17], charcoal[18], and carbon nanotubes (CNTs)[19–21]. In fact, most of them have also been demonstrated to achieve HML. In particular, CNTs are intensively applied SAs that process favorable properties of a fast recovery time, wideband wavelength range, as well as being insensitive to environmental perturbation[22]. Moreover, in order to improve the damage threshold and emit higher energy, a CNT SA of the evanescent-wave interaction type is proposed, which is formed by deposition on a D-shaped fiber[23], a fiber taper[24], or a microfiber[25]. Jun et al. first obtained a 943 MHz pulse train at the 34th harmonics with excellent noise performance under 195 mW pump power[26]. Furthermore, through engineering the dispersion of the cavity, 4.9 GHz pulses, corresponding to the 128th HML was finally realized under 395 mW pump power with 40 dB super-mode suppression ratio (SMSR)[27]. However, the structure is considerably conditional on the special fiber devices, which will bring additional complexity to the system[28]. Another credible SA type is a CNTs film sandwiched between two fiber connector ferrules, exhibiting virtues of simple construction, mature manufacturing, easy implementation, and high flexibility[29–31]. Nevertheless, the CNTs film is rarely exploited to achieve a relative repetition rate pulse train. Up till now, the highest repetition rate of 328 MHz, corresponding to the 23rd harmonics with a larger than 30 dB SMSR, has been examined by a passively harmonically mode-locked fiber laser using CNTs film[32].
In this work, we experimentally demonstrate passive HML of an erbium-doped fiber laser (EDFL) using a CNTs polyvinyl alcohol (PVA) film. After slightly adjusting the intra-cavity birefringence, the laser is in a position to deliver pulse trains with a 580 MHz repetition rate, corresponding to the 22nd harmonics of the 26.3 MHz fundamental repetition rate under 323 mW pump power. To the best of our knowledge, this is the highest repetition rate achieved by using a CNTs film as an SA. Especially, the radio frequency (RF) spectrum indicating a 40.6 dB SMSR, which is significantly preferred to a prior HML laser reported in Ref. [24]. Also, other systematic performances of the train are depicted. The demonstrated laser system attests that it has potential to generate pulses with a high repetition rate by just using CNTs film by means of HML.
Sign up for Chinese Optics Letters TOC. Get the latest issue of Chinese Optics Letters delivered right to you!Sign up now
The experimental configuration of the proposed CNTs-PVA film-based fiber laser is illustrated in Fig. 1. A 98.2 cm erbium-doped fiber (EDF, Er110-4/125 from Liekki) functions as gain medium, whose signal gain is 110 dB/m at 1530 nm with a normal dispersion of at 1550 nm. A 980 nm benchtop laser (OV LINK, Wuhan, China) with the maximum pump power of 710 mW provides pump light, which is launched into the cavity resonator through a wavelength-division multiplexer (WDM) coupler. A 55.8 cm OFS 980, which is the pigtail of the WDM coupler, offers group velocity dispersion (GVD) of . The 20% pulse energy is tapped out with an optical coupler (OC). In order to transmit light unidirectionally, one polarization independent isolator (ISO) is employed, while a polarization controller (PC) is placed beside the ISO to control the cavity polarization. The CNTs-PVA film is incorporated into the resonator by sandwiching it between two fiber connectors directly serving as the SA. The cavity length is 7.91 m, which corresponds to a 26.3 MHz fundamental repetition rate, including the 153.3 cm HI 1060 with a GVD coefficient of and a 470.6 cm standard single mode fiber (SMF) with a GVD coefficient of . Therefore, the total dispersion is , which leads to laser operation in the soliton regime. The characteristics of the output beam are recorded by an optical spectrum analyzer (OSA, Yokogawa AQ6370C), a 1 GHz oscilloscope (OSC, Tektronix MSO4104) with a 12.5 GHz photo-detector (PD, Newport 818-BB-51F), and an RF spectrum analyzer (SIGLENT, SSA 3032X) together with a commercial autocorrelator (FEMTOCHROME, FR-103WS).
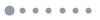
Figure 1.Schematic configuration of a passive HML fiber laser with a CNTs-PVA film.
The production process of the CNTs-PVA film is similar to Ref. [25]. The linear optical absorption spectrum of the CNTs-PVA film is depicted in Fig. 2(a), which gives a broad absorption band, and the absorbance is nearly 0.2 at 1550 nm. Figure 2(b) is the measured Raman spectrum with an excitation wavelength of 532 nm. Because the radial breathing mode (RBM) is in relation to the diameter of CNTs[33], we can calculate a mean nanotube diameter of 0.88 nm, according to a RBM. Moreover, the existence of the RBM and G mode () suggests the CNTs are single walled. We have also found that the D mode is hard to detect, declaring high quality of the CNTs sample. Figure 2(c) shows the measured nonlinear transmission, from which the modulation depth can be estimated as about 6.2%, which gives full proof that the CNTs-PVA film has the capability to be served as an ideal mode locker.
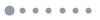
Figure 2.(a) Absorption spectrum of the CNTs-PVA film. (b) Measured Raman spectrum of the CNTs-PVA film under a pump laser of 532 nm. (c) The measured nonlinear transmission of the CNTs-PVA film.
Mode-locked operation occurs when the pump power reaches 88 mW with proper adjustment of the PC. Figure 3 presents some characteristics of pulses under 95.6 mW pump power. The RF spectrum illustrated in Fig. 3(a) shows that the laser works in a single pulse state with a 26.3 MHz fundamental repetition rate, which quite agrees with a 7.91 m cavity length, while the inset of Fig. 3(a) shows that a 3 dB bandwidth of the pulses is 5.8 nm with a 1563.48 nm center wavelength. Also, the existence of the Kelly sideband confirms that the laser operates in the soliton regime. Figure 3(b) is the corresponding OSC image, from which it is apparent that the envelope of the mode-locked pulse train consists of a tiny peak fluctuation with , suggesting a slightly instable state. The measured output power is 0.328 mW.
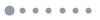
Figure 3.Characteristics of pulses with 26.3 MHz fundamental repetition rate under 95.6 mW pump power: (a) RF spectrum with a 3.2 GHz span and 10 kHz resolution bandwidth. Inset is the corresponding optical spectrum. (b) Oscilloscope trace.
The 2nd–22nd-order HML can be observed in the wake of continually increasing pump power. When 323 mW pump power is applied, the 22nd HML pulses at a 580 MHz repetition rate appear with 4.9 mW output power. The pulse train is shown in Fig. 4, from which we can see that the interval between adjacent pulses is well-distributed and equally spaced. It is measured to be 1.72 ns, indicating a 580 MHz repetition rate.
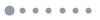
Figure 4.Observed pulse train at 580 MHz repetition rate. Inset presents a pulse train within the range of 0.2 μs.
As is universally known, the RF spectrum plays a great role in evaluating the stability of the laser operation depicted in Fig. 5. The maximum peak in Fig. 5 located in 580 MHz further verifies the repetition rate of the pulses. The SMSR at the level of 40.6 dB prevails over the previous report[32], and the signal-to-noise ratio (SNR) is 48.2 dB. Both of them manifest that the laser possesses good stability. Figure 6 is the corresponding optical spectrum centered at 1560.13 nm with a 2.55 nm bandwidth. The autocorrelation trace, as illustrated in Fig. 7, implies that the pulse duration is 2.17 ps when fitted by a pulse shape. Thus, the time bandwidth product (TBP) is reckoned to be 0.682, indicating a slight pulse chirp. Furthermore, a shorter pulsewidth and more stable pulses may be obtained if we carefully optimize the CNT dopant concentration in the PVA film or adjust the pump power properly[30,34].
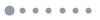
Figure 5.RF spectrum over a 3.2 GHz span of the emitted light from the fiber laser. The RF peak of the insert map is 580 MHz.
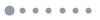
Figure 6.Optical spectrum of the pulses.
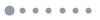
Figure 7.(Color online) Autocorrelation trace of the pulses.
The relation of harmonic order versus pump power is plotted in Fig. 8. As we can see, the harmonic order varies from 2 to 22 when the pump power increases from 95.6 to 323 mW. It is apparent that the trend of the variation of the harmonic order is nearly linear. In our experiment, when the pump power is rising further, -switching will arise instead of the mode-locking operation. Considering the soliton area theorem[35], higher repetition rate pulses are expected to be achieved though appropriately optimizing the laser average dispersion to get less soliton energy[11,21].
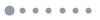
Figure 8.Harmonic order as a function of pump power.
In conclusion, we demonstrate passive HML of an EDFL using CNTs film as an SA. Though increasing pump power and manipulating the PC, 580 MHz repetition rate pulses, corresponding to the 22nd harmonics of the 26.3 MHz fundamental repetition rate, can be realized, which, to the best of our knowledge, are the highest repetition rate pulses generated from an EDFL based on the CNTs film. Particularly, the stability of the pulses is proved by the 40.6 dB SMSR. The laser can work for several hours at a stable operation. Furthermore, our experiment results inspire us to reveal that the CNTs polymer composite film is able to function as an SA applied in a fiber laser to obtain high repetition rate pulses via HML.
References
[1] R. J. Jones, J.-C. Diels. Phys. Rev. Lett., 86, 3288(2001).
[2] H. A. Haus, W. S. Wong. Rev. Mod. Phys., 68, 423(1996).
[3] J. B. Schlager, P. D. Hale, D. L. Franzen. Microwave Opt. Technol. Lett., 6, 835(1993).
[4] W.-W. Hsiang, C.-Y. Lin, M.-F. Tien, Y. Lai. Opt. Lett., 30, 2493(2005).
[5] R. Wang, Y. Dai, F. Yin, K. Xu, L. Yan, J. Li, J. Lin. Opt. Express, 21, 20923(2013).
[6] H. Qi, J. Zhang, G. Zhou, A. Wang, Z. Zhang. Chin. Opt. Lett., 11, 061402(2013).
[7] L. Zhao, C. Lu, H. Tam, P. Wai, D. Tang. IEEE Photon. Technol. Lett., 21, 724(2009).
[8] Y. L. Qi, H. Liu, H. Cui, Y. Q. Huang, Q. Y. Ning, M. Liu, Z. C. Luo, A. P. Luo, W. C. Xu. Opt. Express, 23, 17720(2015).
[9] Y. F. Song, L. Li, H. Zhang, D. Y. Tang, K. P. Loh. Opt. Express, 21, 10010(2013).
[10] D. Tang, L.-M. Zhao, B. Zhao, A. Liu. Phys. Rev. A, 72, 043816(2005).
[11] Z. C. Luo, M. Liu, H. Liu, X. W. Zheng, A. P. Luo, C. J. Zhao, H. Zhang, S. C. Wen, W. C. Xu. Opt. Lett., 38, 5212(2013).
[12] G. Sobon, J. Sotor, K. M. Abramski. Appl. Phys. Lett., 100, 161109(2012).
[13] S. Tao, L. Xu, G. Chen, C. Gu, H. Song. J. Lightwave Technol., 34, 2354(2016).
[14] S. Zhou, D. G. Ouzounov, F. W. Wise. Opt. Lett., 31, 1041(2006).
[15] H. Sotobayashi, C. Sawaguchi, Y. Koyamada, W. Chujo. Opt. Lett., 27, 1555(2002).
[16] Y. Deng, M. Koch, F. Lu, G. W. Wicks, W. H. Knox. Opt. Express, 12, 3872(2004).
[17] G. R. Lin, Y. C. Lin. Laser Phys. Lett., 8, 880(2011).
[18] Y. H. Lin, Y. C. Chi, G. R. Lin. Laser Phys. Lett., 10, 055105(2013).
[19] H. Li, S. Zhang, J. Du, Y. Meng, Y. Hao, X. Li. Opt. Commun., 285, 1347(2012).
[20] C. Mou, R. Arif, A. Rozhin, S. Turitsyn. Opt. Mater. Express, 2, 884(2012).
[21] K.-N. Cheng, Y.-H. Lin, G.-R. Lin. Laser Phys., 23, 045105(2013).
[22] S. Kivistö, T. Hakulinen, A. Kaskela, B. Aitchison, D. P. Brown, A. G. Nasibulin, E. I. Kauppinen, A. Härkönen, O. G. Okhotnikov. Opt. Express, 17, 2358(2009).
[23] Y.-W. Song, S. Yamashita, S. Maruyama. Appl. Phys. Lett., 92, 021115(2008).
[24] Y.-W. Song, K. Morimune, S. Y. Set, S. Yamashita. Appl. Phys. Lett., 90, 021101(2007).
[25] Z. Yu, Y. Wang, X. Zhang, X. Dong, J. Tian, Y. Song. Laser Phys., 24, 015105(2013).
[26] C. S. Jun, J. H. Im, S. H. Yoo, S. Y. Choi, F. Rotermund, D.-I. Yeom, B. Y. Kim. Opt. Express, 19, 19775(2011).
[27] C. S. Jun, S. Y. Choi, F. Rotermund, B. Y. Kim, D.-I. Yeom. Opt. Lett., 37, 1862(2012).
[28] T. Wang, Z. Yan, C. Mou, K. Zhou, L. Zhang. Appl. Opt., 56, 3583(2017).
[29] C. Zou, T. Wang, Z. Yan, Q. Huang, M. AlAraimi, A. Rozhin, C. Mou. Opt. Commun., 406, 151(2018).
[30] K.-N. Cheng, Y.-H. Lin, S. Yamashita, G.-R. Lin. IEEE Photon. J., 4, 1542(2012).
[31] Y.-C. Lin, K.-N. Cheng, G.-R. Lin. 2011 16th Opto-Electronics and Communications Conference (OECC), 788(2011).
[32] K. Jiang, S. Fu, P. Shum, C. Lin. IEEE Photon. Technol. Lett., 22, 754(2010).
[33] D. Kahn, J. P. Lu. Phys. Rev. B, 60, 6535(1999).
[34] J.-C. Chiu, C.-M. Chang, B.-Z. Hsieh, S.-C. Lin, C.-Y. Yeh, G.-R. Lin, C.-K. Lee, J.-J. Lin, W.-H. Cheng. Opt. Express, 19, 4036(2011).
[35] L. Nelson, D. Jones, K. Tamura, H. Haus, E. Ippen. Appl. Phys. B Lasers Opt., 65, 277(1997).