Abstract
We report on the frequency stabilization of Er-doped fiber lasers against rotovibrational absorption lines of molecular at 1550 nm by means of the frequency modulation spectroscopy technique. The laser frequency is locked to the saturated dispersion signal of an HCN cell and reached a stability of for a 100 s integration time without frequency modulation.A variety of frequency stabilization lasers near the 1550-nm transmission window of optical fibers are required not only for frequency standards of wavelength division multiplexed systems, but also for coherent optical communication, optical frequency synthesizers, fiber-optic sensing, laser spectroscopy, and metrology[1–4]. Numerous studies have been devoted to the absolute stabilization of 1550-nm lasers. In order to achieve higher accuracy and stability, locking to narrower transitions was developed by use of weak absorption signals for the frequency stabilization of semiconductor lasers and fiber lasers[5,6]. Of the many available lasers, the fiber laser is a particularly attractive candidate for such fields because of its narrow linewidth.
In this Letter, absolute frequency stabilization of an ultra-narrow linewidth ring-cavity fiber laser around 1550 nm is reported. The frequency of the laser was stabilized to a overtone vibrational absorption line of by using the modulation transfer spectroscope (MTS) method. A fiber Bragg grating (FBG) is fixed on a piezoelectric transducer (PZT) module to lock the laser frequency at the selected absorption line of HCN. With this configuration, we obtained a stable optical output beam with no frequency modulation. The stabilized oscillators show the best frequency stability, for an integration time of 100 s; the Allan variance is less than , and the line width is less than 300 Hz.
A schematic of the frequency-stabilization loop, based on the MTS technique, is shown in Fig. 1. The laser has two main parts. One is a tunable, polarization maintained single-frequency fiber ring laser. The other is a laser frequency stabilization unit. A small fraction (about 10%) of the output beam is transmitted into the laser frequency controller by a fiber coupler as the frequency-stabilization beam. The frequency-stabilization beam is further split into two parts: the pump beam is phase modulated by means of an electro-optic modulator at 10 MHz with a phase index of 0.85. To reduce the optical feedback at the photodetector, the unmodulated probe beam is frequency shifted by 80 MHz using an acoustic-optic modulator. The pump and probe beams are counter-propagating with orthogonal polarizations through the 8-cm long HCN cell with powers of approximately 3 mW. With these polarization directions the re-emitted beams (four-wave mixing effect) combine with the probe at the photodetector to produce MTS lineshape signals. The double balanced mixer (DBM) in Fig. 1 generates an error signal that is proportional to the frequency deviation. The error signal after passing through a PZT driver is sent back to the PZT, and a FBG in the laser cavity is attached on the PZT to control the oscillation frequency of the fiber laser. In order to improve the temperature characteristics of the frequency tuning, both of them are put in a sealed thermotank. By applying a voltage signal to the PZT, the laser wavelength was continuously tuned over 0.1 nm without mode hopping. Figure 2 shows the tuning characteristic of the fiber laser.
Sign up for Chinese Optics Letters TOC. Get the latest issue of Chinese Optics Letters delivered right to you!Sign up now
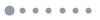
Figure 1.Configuration of frequency-stabilized fiber laser.
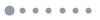
Figure 2.Tuning characteristic of our fiber laser.
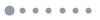
Figure 3.Output of the lock-in amplifier measured while varying the lasing frequency.
The saturated P(11) line of the overtone vibrational of has been selected as a frequency reference at 1550 nm to lock the laser frequency against the resonance with the Doppler-free spectroscopy method[7,8]. The calculated vacuum wavelength of the P(11) line center is 1550.5149 with an expanded uncertainty of through low pressure.
The corresponding modulation transfer signal is measured with our new PSD circuit as plotted in Fig. 3. The signal shows the magnitude and direction of the frequency shift from the center frequency of the linear absorption line. Frequency stabilization of the fiber laser was achieved by feedback control using the observed modulation transfer signal.
Frequency stability was evaluated by observing the beat-note frequency between two independent but identical laser systems[2,9]. Figure 4 shows the square root Allan variance of the observed beat-note when the lasers were locked. The laser stability reached a level of when the integration time was larger than 100 s.
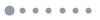
Figure 4.Square root of the Allan variance of the frequency fluctuation of our frequency-stabilized fiber laser.
The linewidth of frequency-stabilized fiber laser was measured using a self-heterodyne interferometric technique. The power spectrum was observed for 25 km-delay fiber. Figure 5 shows the electrical spectrum of the heterodyne signal. The full-width half-maximum of the RF spectral width is given. From Fig. 6, we estimated the linewidth of the frequency-stabilized fiber laser to be approximately 0.5 kHz. This result indicates that the present frequency-stabilized fiber laser has an output beam with no frequency modulation, and under a single-frequency condition.
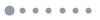
Figure 5.Electrical spectrum of the delayed self-heterodyne signal.
References
[1] M. Marano, P. Laporta, A. Sapia, P. D. Natale. Opt. Lett., 25, 1702(2000).
[2] K. Kasai, M. Yoshida, M. Nakazawa. Optical Fiber Communication Conference and Exposition and The National Fiber Optic Engineers Conference, OWM4(2006).
[3] C. Chou, T. Lin, P. Huang, M. Chien. IEEE Photonics Technol. Lett., 16, 1948(2004).
[4] A. Sträßer, M. Ostermeyer. Conference on Lasers and Electro-Optics/Quantum Electronics and Laser Science Conference and Photonic Applications Systems Technologies, CTuQ1(2008).
[5] P. Laporta, S. Taccheo, S. Longhi, C. Svelto, P. De Natale. Appl. Phys. Lett., 71, 2731(1997).
[6] C. Latrasse, M. Breton, M. Têtu, N. Cyr, R. Roberge, B. Villeneuve. Opt. Lett., 19, 1885(1994).
[7] A. Arie, S. Schiller, E. K. Gustafson, R. L. Byer. Opt. Lett., 17, 1204(1992).
[8] K. Nakano, S. Maehara, M. Yanagisawa, K. Doi, T. Sato, M. Ohkawa, T. Maruyama, S. Kawamura. Proc. SPIE, 6829, 68290R(2007).
[9] R. Guo, F. Hong, A. Onae, Z. Y. Bi, H. Matsumoto. Opt. Lett., 29, 1733(2004).