
- Chinese Optics Letters
- Vol. 13, Issue Suppl., S21403 (2015)
Abstract
Stimulated Raman scattering (SRS) has been shown to be an effective method for wavelength conversion of laser radiation in recent years. Due to various advantages of compactness, high stability, small scale, low loss, and high efficiency, Raman crystals have been widely employed in many fields such as information, communication, medical treatment, and so on. Nd:YVO4 crystals have been considered as an efficient Raman medium in diode-pumped solid-state self-Raman lasers because of its high Raman gain (4.5 cm/GW)[
In 2001, Kaminskii
For a self-Raman laser, thermal effects, caused by the absorption of pump light and the SRS cascading frequency conversion process in the
Sign up for Chinese Optics Letters TOC. Get the latest issue of Chinese Optics Letters delivered right to you!Sign up now
In this work, we demonstrated a diode-end-pumped actively Q-switched
Our experimental setup of a Q-switched third-Stokes self-Raman laser is shown in Fig.
Figure 1.Schematic diagram of the third-Stokes self-Raman laser.
Using the aforementioned experimental configuration described, the average output powers of third-Stokes Raman lasers at 1487 nm versus the incident pump power were measured at different PRFs of 15, 30, and 50 kHz, as shown in Fig.
Figure 2.Average output power versus incident pump power at different PRFs.
A typical optical spectrum of output radiation at an incident pump power of 34 W and a PRF of 30 kHz is shown in Fig.
Figure 3.Optical spectrum of the third-Stokes self-Raman laser.
The line widths at the wavelengths of 1064.4, 1313.4, and 1487.2 nm were measured to be 0.18, 0.32, and 0.175 nm, respectively. The spectrum of the third-Stokes radiation is shown in Fig.
Figure 4.Line width of the third-Stokes self-Raman laser.
A diffraction grating was used to disperse the fundamental, second-, and third-Stokes output radiation. The energy of the first-Stokes radiation was mostly converted to higher-order Stokes. The temporal profiles of the output pulses were recorded by a digital phosphor oscilloscope with a fast photodiode detector at a pump power of 34 W and a PRF of 30 kHz, as shown in Fig.
Figure 5.Temporal profiles of output pulses at a pump power of 34 W and a PRF of 30 kHz.
Finally, the beam quality factor (
In conclusion, a diode-end-pumped actively Q-switched third-Stokes
References
[1] A. A. Kaminskii, K. Ueda, H. J. Eichler, Y. Kuwano, H. Kouta, S. N. Bagaev, T. H. Chyba, J. C. Barnes, G. M. A. Gad, T. Murai, J. Lu. Opt. Commun., 194, 201(2001).
[2] Y. F. Chen. Opt. Lett., 29, 2172(2004).
[3] S. H. Ding, X. Y. Zhang, Q. P. Wang, F. F. Su, P. Jia, S. T. Li, S. Z. Fan, J. Chang, S. S. Zhang, Z. J. Liu. IEEE J. Quantum Electron., 42, 927(2006).
[4] H. Y. Zhu, Y. M. Duan, G. Zhang, C. H. Huang, Y. Wei, H. Y. Shen, Y. Q. Zheng, L. X. Huang, Z. Q. Chen. Opt. Express, 17, 21544(2009).
[5] C. L. Du, L. Zhang, Y. Q. Yu, S. C. Ruan, Y. Y. Guo. Appl. Phys. B, 101, 743(2010).
[6] X. Ding, C. Fan, Q. Sheng, B. Li, X. Y. Yu, G. Z. Zhang, B. Sun, L. Wu, H. Y. Zhang, J. Liu, P. B. Jiang, W. Zhang, C. Zhao, J. Q. Yao. Opt. Express, 22, 29111(2014).
[7] G. X. Huang, Y. Q. Yu, X. H. Xie, Y. F. Zhang, C. L. Du. Opt. Express, 17, 19723(2013).
[8] W. Chen, Y. Wei, C. Huang, X. Wang, H. Shen, S. Zhai, S. Xu, B. Li, Z. Chen, G. Zhang. Opt. Lett., 37, 1968(2012).
[9] C. L. Du, Y. Y. Guo, Y. Q. Yu, G. X. Huang, S. C. Ruan. Laser Phys. Lett., 10, 055802(2013).
[10] C. L. Du, X. H. Xie, Y. F. Zhang, G. X. Huang, Y. Q. Yu, D. D. Wang. Appl. Phys. B, 116, 569(2014).
[11] C. L. Du, X. H. Xie, Y. F. Zhang, G. X. Huang, Y. Q. Yu, D. D. Wang. Laser Phys. Lett., 24, 125003(2014).
[12] H. M. Pask, S. Myers, J. A. Piper, J. Richards, T. McKay. Opt. Lett., 28, 435(2003).
[13] Y. T. Chang, Y. P. Huang, K. W. Su, Y. F. Chen. Opt. Express, 16, 21155(2008).
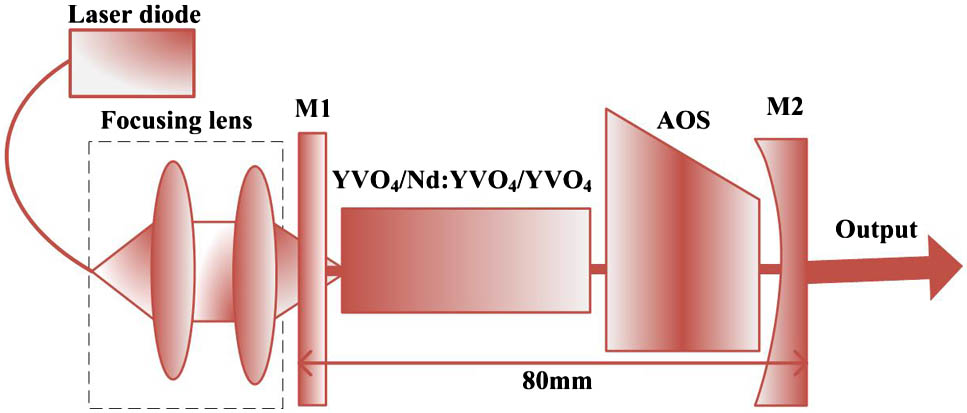
Set citation alerts for the article
Please enter your email address