S. J. Tan1、2、3、*, Z. C. Tiu2、3, S. W. Harun2、3, and H. Ahmad3
Author Affiliations
1School of Engineering, KDU University College, Glenmarie Campus, Jalan Kontraktor U1/14, Seksyen U1, 40150 Shah Alam, Selangor Darul Ehsan, Malaysia2Department of Electrical Engineering, Faculty of Engineering, University of Malaya, 50603 Kuala Lumpur, Malaysia3Photonics Research Center, University of Malaya, 50603 Kuala Lumpur, Malaysiashow less
Abstract
A sideband-controllable soliton mode-locked erbium-doped fiber laser is successfully demonstrated utilizing the nonlinear polarization rotation technique. The sidebands can be produced or suppressed by performing simple polarization light tuning with a polarization controller. It is believed that the elimination of the sidebands is due to the dispersive waves that are filtered out by the polarization-dependent isolator in the resonator. With the elimination of the Kelly sidebands, the obtained 3 dB bandwidth is 10.6 nm and the attainable pulse duration is 0.86 ps. In this experiment, it is proven that the existence of Kelly sidebands limits the attainable pulse duration.In the past few years, bismuth erbium-doped fibers (Bi-EDFs) have been extensively explored for optical amplification[1,2] and fiber laser[3] applications. This fiber incorporates lanthanum (La) ions to decrease the concentration quenching of the erbium ions in the fiber, which allows the erbium ion concentration to be increased to more than 3000 ppm. A fiber with such a high erbium dopant concentration is expected to have enormous potential in realizing compact EDF amplifiers (EDFAs) and EDFA-based devices, such as fiber lasers. The Bi-EDF also exhibits very high fiber nonlinearity, which is useful in some fiber laser applications. For instance, Harun et al.[4] demonstrated a compact Brillouin fiber laser using a piece of 49 cm Bi-EDF as both a linear and nonlinear gain medium.
On the other hand, a soliton pulse is generated by constructing a negative net dispersion cavity[5]. A typical soliton pulse is always accompanied by Kelly sidebands, which appear like sharp spikes distributed on the soliton spectrum. The soliton pulse circulating in the laser cavity periodically endures perturbations such as the gain-provided EDF and loss at the output coupler. The perturbed soliton sheds dispersive radiation as it is reshaped back into a soliton. The constructive interference between the soliton pulse and dispersive waves gives rise to sideband generation, which is then imposed on the soliton spectrum. The positions of the sidebands can be used to estimate the total cavity length, net dispersion, and pulse width[6]. However, the sharp sideband generation puts a limit on the achievable pulse duration and energy[7]. For that reason, it would be desirable if the sidebands could be controlled and subsequently produce a dual-function laser, which can be used to deliver high-quality optical pulses as well as measure the dispersion if the dispersion value of a cavity is unknown.
To date, several methods have been proposed to suppress sideband generation. One of the methods involves constructing a short cavity design, where the Kelly sidebands can be shifted out from the gain bandwidth of the erbium window[6]. Secondly, Kelly sidebands can be suppressed by implementing a hybrid mode-locking scheme, which combines nonlinear polarization rotation (NPR) with the saturable absorption effect[8]. Kelly sideband filtering was demonstrated by incorporating a broad birefriengent plate filter in the laser cavity[9] and also with chirped fiber Bragg gratings[10]. All of the abovementioned techniques can only suppress the sidebands, but do not offer the flexibility to control the sideband generation or suppression.
Sign up for Chinese Optics Letters TOC. Get the latest issue of Chinese Optics Letters delivered right to you!Sign up now
In this Letter, a sideband-controllable passively mode-locked bismuth-based EDF laser (EDFL) is demonstrated with the NPR technique. The generation and elimination of sidebands is clearly observed by performing simple polarization light tuning. After the suppression of the sidebands is achieved, the soliton pulse has a 3 dB bandwidth of 10.6 nm and the obtained pulse duration (assuming that it is fitted to a fitting) is about 0.86 ps. The 3 dB bandwidth is about two times broader, while the pulse width is narrower compared to the soliton pulse with Kelly sidebands. It is believed that the sideband suppression in the fiber laser is the result of the dispersive waves that are filtered by the polarizer in the laser cavity. This is the first demonstration of a sideband-controllable soliton mode-locked fiber laser operating in the picosecond region using such a short length of Bi-EDF.
The experimental setup of the proposed mode-locked fiber laser is schematically shown in Fig. 1. The gain medium used is a piece of 49 cm-long Bi-EDF, which has an erbium ion concentration of 3250 ppm, a cut-off wavelength of 1440 nm, a pump absorption rate of 130 dB/m at 1480 nm, and a dispersion parameter of 130 ps/(km nm) at . Bi-EDFs with such a high erbium dopant concentration exhibit high nonlinearity, making them suitable to be used as a gain medium to generate pulses with the NPR technique. The Bi-EDF is pumped with a 1480 nm laser diode through a 1480 nm laser diode through a 1480/1550 nm wavelength-division multiplexer (WDM). A 1480 nm pump is used rather than a 980 nm pump, as it had been proved that the signal gain generated by the 1480 nm pump is higher than that produced by the 980 nm pump[11]. The gain of the Bi-EDFA varied between 18–23 dB in the C-band region and 5 to 16 dB in the L-band region with an input signal power of 30 dBm, while the noise figure varied between 7–10 dB for wavelength ranging from 1525–1520 nm[12]. A polarization controller (PC) is used to adjust the polarization of light, while a polarization-dependent isolator (PDI) is used to ensure the unidirectional propagation of light in the cavity and at the same time to generate linear light polarization. Two 3 dB couplers are used to extract some portion of light and put light into an optical spectrum analyzer, an oscilloscope, and an autocorrelator for monitoring. The total cavity length is approximately 18 m: 49 cm of Bi-EDF with an angle spliced to 2 m of a single-mode fiber (SMF) at both ends of the Bi-EDF to reduce spurious reflections, 4 m of SMF for the PC, 3 m of SMF for the PDI, 3 m of SMF for the 3 dB coupler, and 3.5 m of SMF for the WDM. The dispersion coefficient for the SMF is 17 ps/(nm km), with an estimated average group velocity dispersion of . The laser cavity runs in the negative dispersion region.
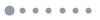
Figure 1.Experimental setup of the sidebands-controllable soliton EDFL with a 49 cm long Bi-EDF as the gain medium.
In the proposed setup, the combination of PC, PDI, and birefriengent fiber acts as an artificial saturable absorber to generate a pulse. This NPR technique is still actively undertaken even with the emergence of new saturable absorbers, such as carbon nanotubes[13] and graphene[14]. This technique offers many advantages, such as fast variable saturable absorption strength and excellent flexibility, and can withstand higher powers compared to the currently available saturable absorbers. When the pump power is increased above the mode-locking threshold and by the proper adjustment of the PC, the fiber laser could easily achieve the mode-locking state. The threshold pump power to achieve the conventional soliton pulse with Kelly sidebands is about 59 mW. The experiment is conducted in a laboratory environment, with the temperature around 20°C. Even though the temperature varies from 18°C to 25°C, mode locking is still achievable when the pump power is set to about 59 mW. The measured optical power (input signal) to sustain mode locking is about 0.8 mW. As the pump power increases to 155 mW and without disturbing the orientation of the PC, the conventional soliton pulse peaks at 1561.3 nm, with a peak output power of and a 3 dB bandwidth of 4.7 nm, as shown in Fig. 2 by the solid line. Generally, Kelly sidebands will be imposed over a broad spectrum of the soliton pulse when the condition for phase matching is satisfied. This happens when the phase difference between the soliton pulse and dispersive waves at the specific frequency is a multiple of , where the soliton pulse interferes constructively with the dispersive waves[7]. It can be seen that the peak power of the Kelly sidebands decreases as the order of the sidebands increases. Higher-order sidebands have a lower peak power, since they are located further away from the maximum gain bandwidth of the laser. The Kelly sidebands on the right of the center wavelength are observed to attain a higher peak power compared to the sidebands on the left. Sidebands asymmetry occurs due to the gain profile amplification in the longer wavelength by the gain peak of the EDF. The amplification process could be also assisted by the Raman effect, where the longer-wavelength sidebands are amplified by the shorter-wavelength solitons[15].
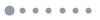
Figure 2.Different spectra profile of the EDFL-based Bi-EDF generated by adjusting the PC.
At the optimum orientation of the PC while maintaining the pump power at 155 mW, the sidebands are completely eliminated. The output spectrum is shown in Fig. 2, represented by the dashed line. In the case of this soliton, which has no Kelly sidebands, the 3 dB bandwidth is 10.6 nm, centered at 1560 nm. The bandwidth is about two times broader than that of the conventional soliton with Kelly sidebands. However, the peak power of the new soliton is slightly lower due to the spectrum broadening effect. The first-order Kelly sidebands (Kelly sidebands that are nearest to the center of the spectrum) are located at 1554.2 and 1568.6 nm at a distance of 7.1 and 7.3 nm away from the center wavelength, respectively. The positions of the Kelly sidebands change when the cavity length is varied. The elimination of the Kelly sidebands can be explained as follows: in the soliton mode-locking state, both soliton and dispersive waves co-propagate in the laser cavity. Since the intensity of the soliton is higher than that of the dispersive waves, only the nonlinear phase delay of the soliton is significant, while the nonlinear phase delay of the dispersive waves can be neglected. The polarization state of the soliton changes due to the Kerr effect as the soliton pulse propagates down the birefringent fiber. With a fixed pump power, the polarization state can also be varied by rotating the PC. Hence, the nonlinear phase delay, which the soliton pulse accumulates in one round trip, differs with the adjustment of the PC. In this experiment, the PC is adjusted in such a way that the accumulated phase difference between the soliton and the dispersive waves is orthogonal upon reaching the PDI. Therefore, only the soliton pulse passes through the PDI, but the dispersive waves are filtered by the PDI. This disturbs the phase-matching condition of the sideband generation. As a result, the spectral sidebands can be suppressed. The sidebands can be reproduced if the PC is rotated in a contrary direction.
The typical mode-locking pulse trains for solitons with and without sidebands are shown in Fig. 3. The fundamental pulse repetition rate for both solitons is 11.3 MHz, which corresponds to the cavity length of about 18 m. Both of the pulse trains are stable in this experiment. Figure 4 represents the autocorrelation trace for both soliton pulses. The estimated pulse duration at its full width at half- maximum is 1.16 and 0.86 ps for the solitons with and without sidebands, respectively. This reveals that the achievable pulse width is limited by the existence of the Kelly sidebands, and the pulse width is successfully narrowed down by filtering out the Kelly sidebands. In addition, the soliton autocorrelation shows an exponentially decaying pedestal due to dispersive wave radiation and the beating of the first- and second-order sidebands[7]. The estimated time bandwidth products (TBPs) corresponding to the shortest pulse widths are 0.67 and 1.12, which are larger than the transform-limited prediction of 0.315 for profiles. This indicates that both pulses are chirped. The TBP indicates how close a pulse is to the transform limit.
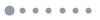
Figure 3.Repetition rate for both pulses at 11.3 MHz.
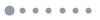
Figure 4.Pulse width measurement for both pulses of the EDFL-based Bi-EDF.
In conclusion, controllable sidebands of a soliton mode-locked EDFL are successfully demonstrated utilizing the NPR technique. When the Kelly sidebands are eliminated from the soliton spectrum by rotating the PC, the obtained 3 dB bandwidth broadens to 10.6 nm, while the pulse duration shortens to 0.86 ps compared to the conventional soliton with Kelly sidebands. The removal of the Kelly sidebands is due to the dispersive waves that are filtered out by the PDI by careful adjustment of the PC. The Kelly sidebands can be brought back by rotating the PC in the contrary direction.
References
[1] X. S. Cheng, B. A. Hamida, A. W. Naji, H. Arof, H. Ahmad, S. W. Harun. IET Optoelectron., 6, 127(2012).
[2] X. S. Cheng, R. Parvizi, H. Ahmad, S. W. Harun. IEEE Photon. J., 1, 259(2009).
[3] A. M. R. Salem, M. H. Al-Mansoori, H. Hizam, S. B. M. Noor, M. A. Mahdi. Laser Phys., 21, 389(2011).
[4] S. W. Harun, R. Parvizi, S. Shahi, H. Ahmad. IEEE Photon J., 1, 254(2009).
[5] C. Zeng, X. Liu, L. Yun. Opt. Express, 21, 18937(2013).
[6] J. Peng, L. Zhan, Z. Gu, K. Qian, S. Luo, Q. Shen. Opt. Commun., 285, 731(2012).
[7] L. E. Nelson, D. J. Jones, K. Tamura, H. A. Haus, E. P. Ippen. Appl. Phys. B: Lasers Opt., 65, 277(1997).
[8] S. Kim, Y. Kim, J. Park, S. Han, S. Park, Y.-J. Kim, S. W. Kim. Opt. Express, 20, 15054(2012).
[9] K. Tamura, C. R. Doerr, H. A. Haus, E. P. Ippen. J. IEEE Photon. Technol. Lett., 6, 697(1994).
[10] D. Han, X. Liu. Opt. Express, 20, 27045(2012).
[11] M. Jung, Y. M. Chang, J. H. Lee. OFC/NF)EC Technical Digest, JW2A.23(2012).
[12] R. Parvizi, S. D. Emami, S. Shahi, S. W. Harun, H. Ahmad. Optoelectron. Adv. Mater. Rapid Commun., 3, 971(2009).
[13] C. S. Jun, S. H. Yoo, S. Y. Choi, F. Rotermund, D. I. Yeom, B. Y. Kim. Opt. Express, 19, 19775(2011).
[14] J. Sotor, G. Sobon, K. Krzempek, K. M. Abramski. Opt. Commun., 285, 3174(2012).
[15] W. S. Man, H. Y. Tam, M. S. Demokan, P. K. A. Wai, D. Y. Tang. JOSA B, 17, 28(2000).