Abstract
We investigate the one/two-photon fluorescence of two synthesized phenoxy-phthalocyanines (Pc1 and Pc2) using the mild reaction coordination method and the nonlinear optical properties of Pc1 and Pc2 in solution are investigated using the Z-scan technique at 800 with a 100 fs pulse width. The results show that both phthalocyanines indicate strong three-photon absorption, and the critical intensity value of Pc2 is higher than that of Pc1 when the contribution of the high excited-state absorption is introduced in the sample. Furthermore, the redshift of one- and two-photon fluorescence spectra can be explained by the reabsorption effect of the molecules. With good solubility and excellent nonlinear optical properties, the samples are expected to be a potential candidate for optical applications and photodynamic therapy.The phthalocyanines (Pcs) and metallophthalocyanines (MPcs) have been studied a great deal for many years[1]. Owing to their high stability under intense heat and light, their diverse molecular structure, easily coordinating with metal elements, and their unique 18 -electron delocalization system, these compound materials exhibit large nonlinear susceptibilities, which lead to their potential applications in a variety of new technology fields, including nonlinear optics[2], electrochromic display devices[3], liquid crystals[4], and so on. For nonlinear optical applications, Pcs are the promising nonlinear optical material due to their small dielectric constants, fast response times, easily integrating into optical components, and intense absorption in the near-IR region[5–7], which lead to potential applications in photonics[8,9], data processing[10], optical switching[11], optical limiting[12,13], and photodynamic therapy (PDT)[14,15]. Furthermore, the large nonlinear optical response of a MPc, arising from its two-dimensional (2D) conjugated -electrons system, lends MPc as a candidate in various photonics devices. Thus, the nonlinear optical materials modified from Pc are increasingly being developed and researched.
In this Letter, we studied the fluorescence properties for two Pcs with phenoxy-tribranched structures. In addition, we investigated the nonlinear optical properties of Pc1 and Pc2 using the Z-scan technique with an 800 nm, 100 fs pulse laser. Our studies in the femtosecond domain provide sufficient evidence that these molecules possess superior nonlinear optical performance for their potential applications.
The Pc1 and Pc2 are hydrogen or zinc connected with four ligands by nitrogen bridges. Specifically, Pc1 and Pc2 are 2D large molecules[16], and their molecular weights are 883u and 946u, respectively. The proton nuclear magnetic resonance of the two synthesized Pcs compounds has been reported by Ref. [17]. The results show that the number and relative intensities of the peaks in the spectra and splittings are identical with the target product (Pc1, Pc2) structure. They have been presented in Fig. 1(a).
Sign up for Chinese Optics Letters TOC. Get the latest issue of Chinese Optics Letters delivered right to you!Sign up now
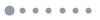
Figure 1.(a) Synthesis and (b) the linear absorption spectra of Pc compounds.
All the experiments were performed in an N,N-dimethylformamide (DMF) dissolved samples solution with a concentration of . The absorption spectra of Pc1 and Pc2 in a ground state () are shown in Fig. 1(b). For both Pcs there are two strong broad bands: the B-band () in the near-UV range ( and 298 nm for Pc1 and Pc2, respectively) and the Q-band () in the red range ( for Pc1 and 704 nm for Pc2). To get a deeper insight into the mechanisms of energy dissipation in Pcs, we have monitored the excited-state dynamics in DMF solvents upon excitation at 800 nm, which promotes the transition in the Q band. The pumping at 400 nm excites the transition and/or the state, as the inset shows in Fig. 1(b).
Figure 2 shows one-photon fluorescence (OPF) spectra of the molecules in DMF. The fluorescence spectra are mirror images of the absorption spectra. The small Stokes shifts suggest that the structural change between the ground and excited states are small. However, the fluorescence spectrum is slightly broader compared to the absorption spectrum. There is a strong fluorescence band in the 700–720 nm regions. The observed fluorescence band remains the same when the excitation wavelength is changed from 360 to 800 nm, which indicates that this observed fluorescence mainly originates from the state even when the state is initially populated following excitation at 400 nm. This result suggests that the state is very short-lived relative to the state and the conversion from to is a rapid process.
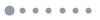
Figure 2.Fluorescence emission spectra of (a) Pc1 and (b) Pc2.
Under the excitation of 800 nm laser pulses with a pulse duration of 100 fs, Pc1 and Pc2 solutions emit the intense frequency upconverted fluorescence. Figure 3 shows two-photon fluorescence (TPF) spectra of two molecules under different intensities of a pump laser. The linear dependence of fluorescence intensity on the square of the excitation intensity shown in the inset can further prove that the fluorescence excited by 800 nm pulses really comes from the two-photon absorption process. In comparison with OPF spectra, the peak wavelengths redshifted for about 10–20 nm. The fluorescence peaks are located at 720 and 740 nm for Pc1 and Pc2, respectively. The redshift can be explained by the reabsorption effect of molecules, and related to the dipole moment of the ground states, the excited states, and the fluorescence emission states, which strong depend on the solvent with high concentration in the TPF experiment. For the low-excitation intensity, there is nearly no one-photon absorption at 800 nm and the sum of two incident photon energies falls into the absorption band. The observed fluorescence emission should be reasonably generated from two-photon excitation. It is known that Pc with open-shell metal atoms generally have short excited-state lifetimes, due to the rapid charge transfer from the excited porphyrin to the metal atoms. For Pc2, there is still weak emission due to the lack of this acceleration. The nonlinear absorption (NLA) may be enhanced by the four peripheral substituents, serving as electron donors along with a central metal atom to form the structure, which can significantly increase the two-photon absorption cross section[15]. When the light intensity of the laser is , the values of the fluorescent intensity for Pc1 and Pc2 are near 1500 and 1900 (arbitrary units), respectively. It indicates that the two-photon absorption ability of Pc2 is significantly stronger than that of Pc1.
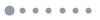
Figure 3.TPF spectra of (a) Pc1 and (b) Pc2 in the DMF under different laser intensities at 800 nm. The power dependencies of the TPF intensity are given in the insets.
Figure 4 shows the open-aperture Z-scans obtained at the same concentration for Pc1 and Pc2 under a femtosecond laser pulse. The experiment was performed at peak intensities. The value of the NLA coefficient was evaluated from the fits to the experimental data obtained using the general equation for open-aperture normalized transmittance. Obviously, the NLA process involved is certainly three-photon absorption (). The estimated values for Pc1 and Pc2 are and , respectively. The dependence of on the different intensities for the samples is depicted in the insets in Fig. 4. We find that remains constant. Clearly, both Pc1 and Pc2 exhibit a strong linear absorption band with peaks at and 298 nm and are highly transparent in the near-IR range. Therefore, one may choose the excitation wavelength () to be 800 nm for Pc1 and Pc2 in order to fulfill the requirements () of three-photon absorption studies. The two-photon absorption dominant NLA must reach until there are sufficient populations in , so that another incident photon can be absorbed and excited from to a higher excited singlet state. The key molecule illustrated by the intensity threshold must be populated to a higher excited singlet state and then absorbed by the two-photon absorption-induced excited saturable absorption process. Then, a nonlinear fifth-order effect based on the highly excited state absorption occurs.
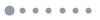
Figure 4.Normalized transmittance curve of femtosecond open-aperture Z-scan curve of (a) Pc1 and (b) Pc2, respectively.
For the femtosecond laser, the simulation results of Pc1 and Pc2 are given in Fig. 5, which illustrates the curves of populations , , , and of the ground state and excited state versus time during a single light pulse under four different excitation intensities. It should be noted that all the molecules will return to the ground state after a sufficiently long time after the pulse. As can be seen from Fig. 5 Pc1(b) and Pc2(b), Pc1 and Pc2 molecules are excited from the ground state to the excited state in the excitation intensity of . Experimental results show that the nonlinear effects stem from the multiphoton absorption of the excited state; in particular, two-photon absorption. We can see from Fig. 5 Pc1(d) and Pc2(d) that most of Pc1 and Pc2 molecules excited from the are further excited to a higher excited singlet state when the excitation intensity is increased to . In this case, a higher excited singlet state of NLA is completely dominated by the highly excited states, and a stronger reverse saturable absorption (RSA) reaction can be seen. In this case, , which can be regarded as a critical population at the critical excitation intensity. It corresponds to the beginning of the transition from saturable absorption to RSA, meaning that the fifth-order effect occurs. We analyze the transient transmission versus time during a light pulse based on the above rate equations. When the input intensity is at , the transmission is decreased with the excitation intensity increasing and increased with the excitation intensity decreasing during the laser pulse. It is an obvious RSA phenomenon. Moreover, the RSA effect becomes stronger with increase of the excitation intensity. Therefore, the theoretical simulation can well elucidate our experimental results. In addition, Fig. 5 shows that the critical value energy of Pc2 is higher than that of Pc1 when the contribution of the high excited-state absorption is achieved in the sample. Since the introduction of the metal zinc will affect the -conjugation of the Pc. The charge transfer will result in the new energy level difference between the HOMO-LUMO and change the nonlinear optical properties of Pc. As a transition metal element there is also the charge transfer mechanism in zinc. But the d-d charge transfer mechanism is not needed to be considered because the solution measurement method is used. In the charge transfer process the electron conjugation levels of or are higher than that of nonmetals. Thus, for the phenoxy Pc derivative, the nonlinear optical performance of the zinc-ion-centered Pc is more excellent than that of the non-ion-centered Pc. The introduction of the peripheral substituents in the Pc will increase the electron density and conjugation degree of the electron of the system, thereby causing an increase in the nonlinear optical parameter values.
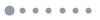
Figure 5.Particle number of Pc1 and Pc2. Intensity is (a) , (b) , (c) , and (d) , respectively.
In conclusion, two Pcs exhibit intense two-photon induced fluorescence under the excitation of femtosecond pulses at a wavelength of 800 nm. In comparison with OPF spectra, their peak wavelengths redshift for about 10–20 nm. The fluorescence peaks are located at 720 and 740 nm for Pc1 and Pc2, respectively. The redshift can be explained by the reabsorption effect of molecules, as we use solutions with a much higher concentration in the TPF experiment. Moreover, using the Z-scan technique, the RSA properties of the two samples are confirmed at a wavelength of 800 nm with femtosecond pulses. The results show that both samples can exhibit three-photon absorption for laser radiation at 800 nm. The NLA coefficient of Pc1 (free base) is smaller than that for Pc2. The Pc and MPc based switches would be potentially useful in optical signal processing and PDT.
References
[1] F. H. Moser, A. L. Thomas. The Phthalocyanines(1983).
[2] Q. Gan, S. Li, F. Morlet-Savary, S. Wang, S. Shen, H. Xu, G. Yang. Opt. Express, 13, 5424(2005).
[3] J. W. Perry, K. Mansour, I. Y. S. Lee, X.-L. Wu, P. V. Bedworth, C.-T. Chen, D. Ng, S. R. Marder, P. Miles, T. Wada, M. Tian, H. Sasabe. Science, 273, 1533(1996).
[4] J. Simon, C. Sirlin. Pure Appl. Chem., 61, 1625(1989).
[5] T. C. Wen, I. D. Lian. Synth. Met., 83, 111(1996).
[6] T. H. Wei, D. J. Hagan, M. J. Sence, E. W. Stryland, J. W. Perry, D. R. Coulter. Appl. Phys. B, 54, 46(1992).
[7] R. S. S. Kumar, S. V. Rao, L. Giribabu, D. N. Rao. Chem. Phys. Lett., 447, 274(2007).
[8] F. Li, P. Lu, H. Long, G. Yang, Y. Li, Q. Zheng. Opt. Express, 16, 1457(2008).
[9] S. V. Rao, N. Venkatram, L. Giribabu, D. N. Rao. J. Appl. Phys., 105, 053109(2009).
[10] P. Gregory. J. Porphyr. Phthalocyan., 4, 432(2000).
[11] P. N. Prasad, D. J. Williams. Introduction to Nonlinear Optical Effects in Molecules and Polymers, 25, 1-199(1991).
[12] S. Tekin, U. Kürüm, M. Durmuş, H. G. Yaglioglu, T. Nyokong, A. Elmali. Opt. Commun., 283, 4749(2010).
[13] Q. Gan, S. Li, F. Morlet-Savary, S. Wang, S. Shen, H. Xu, G. Yang. Opt. Express, 13, 5424(2005).
[14] V. Chauke, M. Durmus, T. Nyokong. J. Photochem. Photobiol. A, 192, 179(2007).
[15] M. Fournier, C. Pépin. Photochem. Photobiol. Sci., 3, 120(2004).
[16] C. B. Yao, Y. D. Zhang, W. J. Sun, C. Q. Yu, J. Li, P. Yuan. Opt. Express, 21, 2212(2013).
[17] Y. D. Zhang, L. Ma, C. B. Yang, P. Yuan. J. Nonlinear Opt. Phys. Mat., 18, 583(2009).