Guang Yong Chu, Adolfo Lerín, Iván N. Cano, Victor Polo, and Josep Prat*
Abstract
In this Letter, an efficient bidirectional differential phase-shift keying (DPSK)—DPSK transmission for a ultra-dense wavelength division-multiplexed passive optical network is proposed. A single distributed feedback laser at the optical network unit (ONU) is used both as the local laser for downlink coherent detection and the optical carrier for uplink. Phase-shift keying is generated using a low-cost reflective semiconductor optical amplifier (RSOA) at the ONU. The RSOA chip has the bandwidth of 4.7 GHz at the maximum input power and bias current. For uplink transmission, the sensitivity of the RSOA chip reaches at the level of bit error for back-to-back, and the penalty for 50 km transmission is less than 1 dB when using polarization diversity.The rapid growth in global telecommunications and in particular, optical fiber communications, has continued to fuel the deployment of fiber access networks that are located closer and closer to the end users[1–3]. Unfortunately, the cost and footprint of the existing access networks are still vital factors to be considered[1–4]. The optical filter at the optical distribution network (ODN) is a large cost consumer for the whole optical fiber network; monolithic integration on the InP is a way to lower the footprint and to facilitate the design of complex photonic circuits with multiple functions[4]. Recently, an integrated semiconductor optical amplifier (SOA) and reflective-SOA (RSOA) have become available for wavelength division-multiplexed passive optical networks (WDM-PONs), reaching 10 Gb/s or more by means of wavelength reusing[5,6] and seeding techniques[7]. Unfortunately, the Rayleigh backscattering (RB) and limited power budget, corresponding to the applications of wavelength reusing and seeding techniques, respectively, can discourage their applications in -agnostic[2] bidirectional ultra-dense WDM-PONs (UDWDM-PONs). Also, WDM-PONs or dense WDM-PON (DWDM-PONs) require optical filtering to select the wavelength for each final user. Furthermore, extending the number of accommodated users requires UDWDM solutions, with the purpose of opening the way to the “wavelength-to-the-user” concept and providing the independent bit rate[2]. With the goal of implementing UDWDM-PONs for the end user, the low-footprint integrated RSOA can be efficiently modulated and coherently detected for the configuration of the access network[8–12].
Considering the power source at the optical network unit (ONU), a typical tunable distributed Bragg reflector laser (DBR) requires more controls, generally implies complex electronics, and has a high cost as compared to the conventional single-electrode distributed feedback laser (DFB)[13]. When using DFB with simple thermal tuning (2 nm) and a statistical initial wavelength distribution of non-preselected ONUs, a record of 256 wavelengths can be allocated in 14 nm with 99.9% availability[14].
Considering the power budget, there is a solution to employ coherent detection and an integrated laser (low footprint) at the ONU to highly enhance the sensitivity and the total bandwidth (BW). At the same time, the phase modulation of the RSOA at the ONU is an advantageous approach for long-reach UDWDM-PONs[9–11].
Sign up for Chinese Optics Letters TOC. Get the latest issue of Chinese Optics Letters delivered right to you!Sign up now
The traditional UDWDM-PON scenario, which uses coherent detection, requires two lasers at the ONU[8,9]. Hence, the stability is dependent on the wavelength drifts of both of the lasers. It is desired that the laser number can be reduced to use only one laser at the ONU in order to reduce the complexity and spectrum overlap[4].
The RSOA-coherent ONU was first demonstrated in Ref. [10] in back-to-back, with the disadvantages of a low modulating BW and a high modulating amplitude requirement. That sentences would be changed as: Unlike that transistor outline can (To-can) RSOA[10], in this Letter, a long cavity and polarization-independent RSOA chip, designed for this application, are used. The RSOA operation is optimized with a modulating BW of 4.7 GHz for the UDWDM-PON. Here, the key benefit of the proposed coherent ONU is that it uses only one single DFB, as a local oscillator (LO) and as a transmitter (Tx) with a phase-modulated RSOA, setting a cost-efficient ONU implementation. Besides, at the optical line terminal (OLT), another DFB is used as the LO in polarization-diversity heterodyne detection without employing any electronic equalization at the receiver (Rx).
The proposed system transmits downstream differential phase-shift keying (DPSK) data at the OLT using coherent detection at the ONU and coherently detects upstream DPSK data at the OLT; the polarization diversity receivers with only two photodetectors (PDs) are exploited for both the ONU and OLT. Both the transmitter and receiver at the ONU can be integrated together in the future, with the purpose of a low-footprint component for the final users. The schematic of the proposed narrow channel spaced access network is shown in Fig. 1. Between downstream and upstream, the wavelength deviation is twice the bit rate for suppressing the RB in the PON.
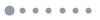
Figure 1.Bidirectional subsystem using heterodyne detection sharing one laser at the ONU for UDWDM-PON using RSOA chip.
With the purpose of validating the proposed schematic given in Fig. 1, the signal spectrum for multi-users bidirectional transmission for access networks is obtained. The two-user DPSK–DPSK optical spectrum in Fig. 2 shows the channel spacing of 12.5 GHz between two users, including down and upstream.
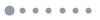
Figure 2.Optical spectrum for 1.25 Gb/s/user DPSK–DPSK bidirectional UDWDM.
An RSOA chip has been implemented with a relatively low optical confinement factor () and a relatively long active section (), which appears to be optimum for this application[10,11,15]. The RSOA has a polarization-independent chip structure, which is discussed in detail in Refs. [16,17], and the test platform is shown in Fig. 3.
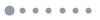
Figure 3.Polarization-independent long-cavity RSOA chip at ONU.
A resistor of 47 Ω is used in series, providing an effective matched impedance, and two wire bonds are used in the sub-mount to increase the modulating BW. The amplified spontaneous emission (ASE) power spectrum of the RSOA chip (biased from 40 to 180 mA, 25°C) displays an optical BW from 20 to 50 nm, as shown in Fig. 4.
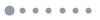
Figure 4.ASE spectrum at different bias condition.
The test fixture of the RSOA chip performs stable coupled output power at room temperature (25°C). The ASE ripple, corresponding to the gain ripple when injecting the optical signal into the RSOA, is influenced by the bias current, the temperature, the reflection, and the wavelength[7]. There is a negligible ripple at 1550 nm around , and the chip can be operated at this wavelength and give a stable performance, as shown in Fig. 4.
The traditional colorless ONU based on the RSOA with wavelength reusing requires the injecting power to be around to . This limits the power budget because of the receiver’s capacity for sensitivity; besides, its 3 dB modulation BW is about 3.5 GHz, as shown in Fig. 5. However, the BW increases to around 4.7 GHz at the higher input power (0 dBm). The bias current and temperature are maintained at 140 mA and 25°C, respectively. It is an optimal condition for BW, and also for reducing the intensity modulation (IM) residual components[7,15], at 25°C. To increase the modulating BW, the RSOA can have more bonding connections added by the manufacturer[18].
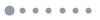
Figure 5.Frequency response as a function of the input powers of 0, , , , , and at the injected current of 140 mA for the RSOA chip.
For the experiments, in order to evaluate the bit error rate (BER) performances, we compare the long-cavity RSOA with a traditional RSOA. The optimal input signal amplitude for the long-cavity 850 μm RSOA chip is and at 1.25 and 2.5 Gb/s, respectively. The To-can packaged RSOA had been equalized using resistor-capacitor (RC) components in order to extend its modulation BW from 800 MHz to 1.5 GHz[5], and its input driving amplitude is 3 V, both for 1.25 and 2.5 Gb/s.
Insensitivity with respect to polarization fluctuations can be achieved in coherent optical communication if the receiver derives two demodulated signals stemming from two orthogonal polarizations of the received signal[19,20]; the schematic is shown in Fig. 6.
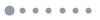
Figure 6.Polarization diversity heterodyne Rx.
A total of encoded bits consisting of non-return-to-zero (NRZ) binary sequences are differentially encoded and modulate the RSOA at 1.25 and 2.5 Gb/s for the external cavity laser (ECL)-DFB and DFB–DFB Tx and LO combinations. The electrical data are amplified before modulation to obtain 180° phase variations, obtaining a DPSK signal. The optical signal, with 0 dBm input power from the DFB at the ONU (5 MHz linewidth) through modulating the RSOA, is then sent back-to-back and after 50 km, comes together with an optical splitter at a remote node for ultra-dense channels. Finally, the optical attenuator is used to change the received input power at the receiver at the OLT.
The OLT receiver for upstream transmission is based on heterodyne detection and composed of a 50/50 coupler that mixes the received signal with the LO. The LO is firstly an ECL (100 kHz linewidth) and secondly a DFB (4 MHz) tuned at the emission frequency offset of 2.5 GHz (for 1.25 Gb/s) and of 5 GHz (for 2.5 Gb/s) above the transmitted wavelength. A polarization beam splitter (PBS) is included to resolve the polarization fluctuations, and only two single-ended PDs[20,21] are used for the polarization diversity detection. The electrical signals are then sampled with a 50 Gsamples/s real-time oscilloscope (DPO71254B, limited at 12.5 GHz). The samples are filtered with a high-pass filter (HPF) after the low pass filtering from the oscilloscope and passed through a 1-bit delay-and-multiply block for differential demodulation (Fig. 6). Before the bit decision, samples are filtered with a fourth-order Butterworth low-pass filter (LPF).
For comparison, the To-can RSOA is mainly used for colorless ONUs, as in our previous work[5]. As explained in the first paragraph, the RSOA is traditionally used as an intensity modulator for WDM-PONs. Here, we modulate the signal in phase and use the polarization diversity receiver to coherently receive the signal without a polarization controller.
First, it is necessary to optimize the receiver in order to obtain the optimal condition for testing. In order to select the optimal operation point of the filters in the digital signal processing (DSP), we measure the BER for different cut-off frequencies for the fourth-order LPF and HPF.
The optimal conditions for the electrical filters at 1.25 Gb/s are shown in Fig. 7. From Fig. 7, it is noted that the LPF and HPF are optimized at 1.0 and 1.4 GHz, respectively.
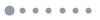
Figure 7.BER penalty against cut-off frequencies of the LPF (Rx input power at ) and HPF (Rx input power at ) at 1.25 Gb/s.
The transmitter source is a DFB whose wavelength is influenced by the temperature controller, and as for the LO, we compare the DFB and ECL. For proper heterodyne detection, the two wavelengths should be precisely matched[4]. We investigate the BER penalty caused by the mismatch around the nominal intermediate frequency (IF); Fig. 8 shows the IF margins of around 50 and 100 MHz that are tolerable at 1.25 and 2.5 Gb/s, respectively.
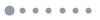
Figure 8.BER penalty versus frequency offset between Tx and LO (Rx input power at and for 1.25 and 2.5 Gb/s, respectively).
At , the measured Rx sensitivities are around and for 1.25 and 2.5 Gb/s, respectively (Fig. 9), by first using the To-can RSOA. After 50 km, and with fluctuating polarization, the power penalty is limited to only 0.5–0.8 dB (from to at 1.25 Gb/s, and from to at 2.5 Gb/s). Next, we apply the DFB as the Tx and another DFB as the LO; the Rx sensitivity is then around for back-to-back and after 50 km at 1.25 Gb/s (Fig. 9). Compared to the DFB-ECL scheme, the two-DFBs scheme presents low cost advantages and only around a 1.5 dB (1.5 dB for back-to-back, 1.6 dB after 50 km) penalty, mostly caused by the increased phase noise and wavelength drifts; the influences of the wavelength drifts can be reduced via automatic wavelength controlling[22].
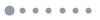
Figure 9.BER against Rx power back-to-back and after 50 km at 1.25 and 2.5 Gb/s (DFB as transmitter at the ONU, and ECL as the LO at the OLT) together with 2 DFBs at 1.25 Gb/s.
Now, using the RSOA chip for upstream modulation, and at the ONU, the DFB laser as the emitter and as the downlink LO, we also test it at 1.25 and 2.5 Gb/s at 25°C. At , the sensitivity reaches (1.25 Gb/s) and (2.5 Gb/s), as shown in Fig. 10. The chip is designed with a relatively low value (), which appears to be the optimum one. In order to compare the performances between the two kinds of chips, the To-can RSOA’s performance in the back-to-back condition are also added again in Fig. 10. Compared with the To-can packaged RSOA, the 850 μm RSOA chip improves around 1 dB of the Rx sensitivity at 1.25 and 2.5 Gb/s (back-to-back, 25°C). The optimal input driving amplitudes are (1.25 Gb/s) and (2.5 Gb/s). These are 10.9 dB (1.25 Gb/s) and 6.2 dB (2.5 Gb/s) lower than the case of the To-can RSOA, which highly benefits the electrical power requirements. The RSOA chip is also tested at the temperature of 22°C, as shown in Fig. 10; for back-to-back transmission, the sensitivity reaches and for 1.25 and 2.5 Gb/s, respectively. There is less than a 1 dB penalty between back-to-back and 50 km transmission. Significantly, the RSOA chip could be integrated together with the laser as an integrated component, which would be a simplified and low-footprint element for the ONU Tx and Rx.
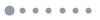
Figure 10.BER versus Rx power using RSOA chip compared with To-can RSOA (back-to-back, 25°C), and RSOA chip at 22°C.
Furthermore, the proposed system provides a total power budget of 52.2 dB for the ODN loss at 1.25 Gb/s, and 48.3 dB at 2.5 Gb/s. It can be exploited to support a large splitting ratio and long-reach PON. This is more than enough to support long-reach operations[23] (up to 100 km length, corresponding nominally to 20 dB losses) and a more than 28 dB[24] margin to allow for power splitting and other intrinsic ODN losses.
A simple, cost-effective, hardware-efficient, and high-sensitivity scheme at the ONU is proposed, increasing the loss budgets and separating the wavelength between the downstream and upstream, which would improve the bidirectional system’s performance significantly. A polarization-insensitive heterodyne receiver is built at the OLT with a minor penalty comparing the ECL and DFB as LOs that can be integrated as a low-footprint and cost-effective transceiver at the ONU[2,12].
The high injected power, unlike the traditional seeded RSOA ONUs, significantly improves the modulating BW and the power budget. Compared with the To-can packaged RSOA, the RSOA chip presents the advantages at the BW, sensitivity, and power consumption. Furthermore, the schematic at the ONU can be integrated as a -agnostic ONU with a wavelength for each user, which is suitable for UDWDM-PON.
By optimizing the receiver in terms of sensitivity and polarization independence and by optimizing the modulator, the work here enables a low-cost UDWDM-PON[24,25] with a high channel number and a power budget in excess of 48 dB.
References
[1] F. Saliou, G. Simon, P. Chanclou, M. Brunero, L. Marazzi, P. Parolari, M. Martinelli, R. Brenot, A. Maho, S. Barbet, G. Gavioli, G. Parladori, S. Gebrewold, J. Leuthold. IEEE J. Lightwave Technol., 33, 1602(2015).
[2] J. Prat, I. Cano, M. Presi, I. Tomkos, D. Klonidis, G. Vall-llosera, R. Brenot, R. Pous, G. Papastergiou, A. Rafel, E. Ciaramella. IEEE J. Lightwave Technol., 34, 783(2016).
[3] J. Fabrega, M. Svaluto Moreolo, F. J. Vilchez, B. R. Rofoee, Y. Ou, N. Amaya, G. Zervas, D. Simeonidou, Y. Yoshida, K. Kitayama. Proceedings of OFC(2014).
[4] G. Y. Chu, I. Cano, V. Polo, C. Kazmierski, R. Brenot, J. Prat. IEEE J. Lightwave Technol., 34, 2042(2016).
[5] M. Omella, I. Papagiannakis, B. Schrenk, D. Klonidis, J. A. Lazaro, A. N. Birbas, J. Kikidis, J. Prat, I. Tomkos. Opt. Express, 17, 5008(2009).
[6] M. Morant, T. Quinlan, S. Walker, R. Llorente. Proceedings of OFC(2011).
[7] J. Prat. Proceedings of ECOC(2010).
[8] I. Tomkos, Y. Miyamoto, G. Wellbrock, P. J. Winzer. IEEE Comm. Mag., 53, 20(2015).
[9] J. Prat, V. Polo, P. Zakynthinos, I. Cano, J. A. Tabares, D. Klonidis, I. Tomkos. Opt. Express, 20, 28758(2012).
[10] G. Y. Chu, A. Lerín, I. N. Cano, V. Polo, J. A. Tabares, J. Prat. Proceedings of ACPC(2014).
[11] G. Y. Chu, V. Polo, A. Lerín, J. Tabares, I. N. Cano, J. Prat. Opt. Commun., 357, 34(2015).
[12] M. Presi, R. Corsini, M. Artiglia, E. Ciaramella. Opt. Express, 23, 22706(2015).
[13] M. Pantouvaki, C. C. Renaud, P. Cannard, M. J. Robertson, R. Gwilliam, A. J. Seeds. IEEE Sel. Topics Quan. Electron., 13, 1112(2007).
[14] V. Sales, J. Segarra, V. Polo, J. Prat. IEEE Photon. Technol. Lett., 27, 257(2015).
[15] G. Y. Chu, A. Lerin, I. Cano, V. Polo, R. Brenot, C. Kazmierski, J. Prat. European Semicondictor Laser Workshop(2014).
[16] C. Caillaud, G. Glastre, F. Lelarge, R. Brenot, S. Bellini, J. Paret, O. Drisse, D. Carpentier, M. Achouche. IEEE Photon. Technol. Lett., 24, 897(2012).
[17] G. de Valicourt, D. Make, J. Landreau, M. Lamponi, G. H. Duan, P. Chanclou, R. Brenot. IEEE Photon. Technol. Lett., 22, 191(2012).
[18] N. Lindenmann, G. Balthasar, D. Hillerkuss, R. Schmogrow, M. Jordan, J. Leuthold, W. Freude, C. Koos. Opt. Express, 20, 17667(2012).
[19] L. G. Kazvoski, S. Benedetto, A. E. Willner. Optical Fiber Communication Systems(1996).
[20] I. Cano, A. Lerín, V. Polo, J. Prat. Proceedings of OFC(2014).
[21] I. Cano, A. Lerín, V. Polo, J. Prat. IEEE Photon. Technol. Lett., 26, 973(2014).
[22] J. Tabares, V. Polo, I. Cano, J. Prat. IEEE Photon. Technol. Lett., 27, 443(2015).
[23] M. Presi, F. Bottoni, R. Corsini, G. Cossu, E. Ciaramella. IEEE Photon. Technol. Lett., 26, 107(2014).
[24] R. Gaudino. Proceedings of OFC(2013).
[25] J. Segarra, V. Sales, V. Polo, J. Prat. Proceedings of ICTON(2015).