J. Alaniz-Baylon1, M. Durán-Sánchez1、2、*, R. I. álvarez-Tamayo3, B. Posada-Ramírez1, M. Bello-Jiménez4, B. Ibarra-Escamilla1, A. A. Castillo-Guzman5, and E. A. Kuzin1
Author Affiliations
1Instituto Nacional de Astrofísica, óptica y Electrónica (INAOE), L. E. Erro 1, Sta. Ma. Tonantzintla, Pue. 72824, Mexico2CONACyT-Instituto Nacional de Astrofísica, óptica y Electrónica, A. P. 51 y 216, Pue. 72000, Mexico3CONACyT—Universidad Autónoma de Nuevo León, Av. Universidad S/N, San Nicolás de los Garza, N. L. 66451, Mexico4Instituto de Investigación en Comunicación óptica, Universidad Autónoma de San Luis Potosí, Av. Karakorum 1470, S. L. P. 78210, Mexico5Universidad Autónoma de Nuevo León, Av. Universidad S/N, San Nicolás de los Garza, N. L. 66451, Mexicoshow less
Abstract
We report the experimental investigation of an all-fiber multi-wavelength passively -switched Er/Yb laser with simultaneous gain-switched pulsed operation by using a thulium-doped fiber as a saturable absorber. Laser emission is obtained in three wavelength regions with central peaks at around 1546?nm, 1561?nm, and 1862?nm. Multi-wavelength emission with separation of approximately 1?nm is obtained around the wavelength regions of 1546?nm and 1561?nm. Stable laser pulses are generated in the pump power range from 3.6?W to 7.3?W.1. INTRODUCTION
Fiber lasers operating in wavelength regions of 1.5-μm and 2-μm have attracted great attention because of their potential applications in many research areas and industries such as medical surgery [1–3], optical communications [4], material processing [5], sensing and lidar [6,7], and spectroscopy [8], among others [9,10]. Among the techniques used to produce a pulsed light emission, -switching is one of the most preferred [11–19]. -switched fiber lasers can be designed to generate high-energy optical pulses with durations in the nanosecond range, which can be obtained by implementing both passive and active methods. In the last decade, passively -switched (PQS) operation of fiber lasers has been achieved by using un-pumped rare-earth-doped and co-doped fibers as fiber saturable absorbers (FSAs). This passive technique allows designing simple all-fiber laser systems [20]. In this technique, the laser configurations maintain advantages of cost-effectiveness, high efficiency, and free maintenance; in addition, no intra-cavity components are needed, which makes them compact and simple. Moreover, in recent years, gain switching (GS) has become a promising effective technique to obtain short high-energy laser pulses. GS laser operation is achieved by laser gain on–off commutation when a modulated pump source is used [21]. Then, the characteristics of the pulsed laser emission can be controlled, since the pulsed pump source directly modulates population inversion in the energy levels of the gain medium [20]. In this regard, -switching lasers, used as pump sources, allow the generation of GS laser pulses to achieve pulsed high-energy light emission in the 2-μm region. Because of the different absorption bands of a Tm-doped fiber (TDF), GS laser emission near the 2-μm waveband can be achieved with a TDF laser (TDFL) with a modulated pump source at different operation wavelengths. Taking advantage of the in-band pump and absorption characteristics of the TDF, fast transition from energy level to the upper laser level can be reached. As a result, the cavity gain is switched on and off almost at the same time as the pump pulse [22], where stable GS laser pulse trains can be obtained, as reported in Refs. [23–26]. GS laser operation has been demonstrated by using a 1.55-μm modulated pump source, from which the shortest GS pulse of 10 ns was obtained in a scheme including a 20-cm-long TDF as the gain medium [23]. Approaches with simultaneous GS and mode-locking (ML) operation in Tm/Ho co-doped fiber laser configurations also have been reported [10,20]. Recently, our research group demonstrated a fiber laser setup with simultaneous PQS and GS operation [27].
In this paper, we experimentally demonstrate simultaneous PQS and GS operations of a fiber laser by using an Er/Yb double-clad fiber (EYDCF) and a TDF within the same linear cavity. Stable PQS pulses in the 1.55-μm wavelength region and GS pulses in the 1.8-μm region are obtained. The TDF acts as an FSA to obtain PQS operation at 1.55-μm and simultaneously as gain medium to obtain GS laser emission in the 1.8 µm wavelength range. Thus, the PQS laser emission acts as a modulated pump source to generate GS laser pulses.
2. EXPERIMENTAL SETUP
Figure 1 shows the experimental setup of the proposed PQS fiber laser with simultaneous GS operation based on a TDF FSA. The gain medium is a 3-m-long EYDCF (Nufern, SM-EYDF-6/125-HE) with core diameter of 6.0 μm, NA of 0.21, and cladding diameter of 125 μm. The EYDCF was pumped by a multi-mode pigtailed laser source at 976 nm through a pump combiner. The maximum pump power launched through the EYDCF was 8 W. The linear cavity was limited on each side by fiber loop mirrors (FLMs), used as reflectors. The FLMs were constructed by interconnecting the output ports of an optical coupler (OC). FLM1 was based on a 50/50 coupler and FLM2 on a 90/10 coupler, with reflectivities of 99% and 36%, respectively, in the 1550 nm wavelength region. With the same couplers in the 1.8-μm wavelength region, the measured reflectivities were 95% and 42%, respectively. A 1.3-m-long TDF segment (CorActive, SCF-TM-8/125) with core diameter of 8 μm, NA of 0.17, and cladding diameter of 125 μm acted as an FSA to obtain PQS EYDCF laser operation and, simultaneously, as a gain medium to achieve GS laser emission in the 1.8-μm wavelength range. The unconnected port of the FLM2 was used as laser output.
Sign up for Photonics Research TOC. Get the latest issue of Photonics Research delivered right to you!Sign up now
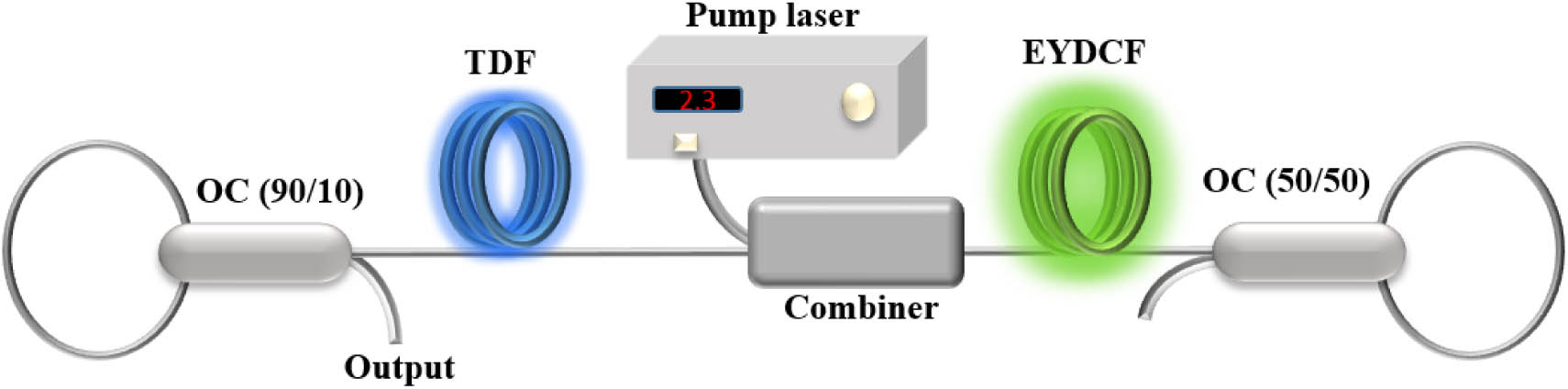
Figure 1.Schematic of the proposed passively -switched and gain-switched fiber laser.
The output laser pulses were detected by two photodiodes, one for the 1.55-μm region (Thorlabs DET01CFC, 1.2-GHz bandwidth and rise/fall time) and the other for the 2-μm region (Thorlabs DET05D, wavelength range 900–2600 nm and 17 ns rise/fall time). The output pulses were monitored by a real-time 2.5-GHz bandwidth oscilloscope. The laser output spectrum was measured by an optical spectrum analyzer (OSA, Yokogawa AQ6375) with scanning range from 1200 nm to 2400 nm and maximal resolution of 50 pm. In order to verify the presence of pulses for multiple generated laser lines in the 2-μm region, a monochromator with resolution of 0.2 nm was used as a wavelength filter.
3. RESULTS AND DISCUSSION
Figure 2(a) shows the setup used to characterize the nonlinear absorption of the TDF-SA. This configuration is based on the average power detection by a balanced twin detector. The input source is a homemade mode-locked erbium-doped fiber laser (MLFL) with central wavelength at 1560 nm, repetition rate of 18 MHz, and pulse duration of 0.5 ps. The MLFL included an amplification stage. A variable optical attenuator (VOA) was used to vary the input optical power from the pump source. The signal from the 10% output port of a 90/10 coupler was used as reference, whereas the 90% coupler port was spliced to TDF-SA. We measured the transmitted power through the TDF-SA. The optical transmittance was obtained as a ratio between average powers at the TDF-SA output and input. The result and the corresponding fitting curve as a function of average pump power are shown in Fig. 2(b). The modulation depth and non-saturable loss were measured as 18% and 37%, respectively.
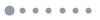
Figure 2.(a) Experimental setup for nonlinear absorption measurement of the TDF-SA and (b) nonlinear characterization of the TDF-SA.
Figure 3 shows the optical spectrum and output laser pulses of the simultaneous PQS and GS laser operation. The measurements were obtained with a pump power of 4.9 W. As can be observed in Fig. 3(a), dual-wavelength -switched laser operation with central wavelengths of 1546 nm and 1561 nm is observed in the 1.55-μm region. Simultaneously, single GS laser emission with a central wavelength of 1862 nm is generated. Zoomed views of both PQS laser wavelengths [Figs. 3(b) and 3(c)] reveal multi-wavelength laser emission of each laser line. For the PQS laser line generated at 1546 nm, four laser lines with central wavelengths at 1544.42 nm, 1545.29 nm, 1546.11 nm, and 1546.98 nm are observed [Fig. 3(b)]. For the laser line at 1561 nm, four laser emissions at 1559.56 nm, 1560.35 nm, 1561.14 nm, and 1561.89 nm are observed [Fig. 3(c)]. We attribute the generation of multiple wavelengths to the filtering effect of the multimode 1550 nm TDF spliced between single-mode fibers. Light entering from single-mode to multimode fiber can excite several modes in the multimode fiber, where the modes travel with different wavenumbers and come to the end of the multimode fiber with different phases. The efficiency of the light coupling from the multimode fiber to the single-mode depends on the relation between phases, resulting in the comb-like dependence of the transmission on the wavelength. TDF (core radius of 4 μm and core NA of 0.17) supports multiple modes at 1.55 μm, as can be evaluated by its calculated normalized frequency of 2.756. Figure 3(d) shows the output spectrum measured at the output port with pump power of 350 mW (below the lasing threshold). The comb output spectrum with a wavelength period of is observed. This value corresponds well with the calculated value given by [28], where is the lasing wavelength, and is the length of the TDF. The laser pulses of the multi-wavelength EYDCF PQS and the single-wavelength TFD GS laser emission are shown in Fig. 3(e). We use for pumping of Tm ions the transition from the base level to the first level . The lifetime of the first level is of [29,30], which is much shorter than the lifetime of Er ions, allowing giant pulse generation with periods of tens of microseconds. The measured pulses were obtained with a pump power of 4.9 W by using two different InGaAs photodetectors, one optimized for the 1.5-μm region and other for the 2-μm region. With the 1.5-μm photodetector (red line), a four-pulse PQS laser regime, which corresponds to the four laser lines generated around 1546 nm, is observed [see Fig. 3(b)]. The four pulses corresponding to the four laser lines at 1560 nm [see Fig. 3(c)] overlap in the fourth pulse, at which they are synchronized. By comparing this measurement with that obtained by using a 2-μm photodetector (blue line), the pulse generated by the GS is observed as a fifth pulse.
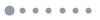
Figure 3.Optical spectra and pulses of the QS and GS fiber laser. (a) Spectrum of EYDCF laser QS centered at 1546 nm and 1561 nm and GS at 1862 nm. (b) Corresponding multi-wavelength first laser line. (c) Corresponding multi-wavelength second laser line. (d) Transmission through the multi-mode TDF to single-mode fiber arrangement. (e) Trains of pulses measured with two photodetectors.
In order to verify the generated PQS pulses corresponding to each multi-wavelength laser line, a monochromator was used as a wavelength filter. Figure 4 shows the pulses corresponding to filtered wavelengths of 1544.42 nm, 1559.56 nm, and 1862 nm. The results were obtained with a pump power of 4.9 W. Single-shot measurements were obtained at the output of the monochromator. In order to compare, the filtered results (red line) were overlapped with unfiltered measurements (blue line) as reference. Figure 4(a) shows the laser pulses for the laser line at 1544.42 nm. A single pulse emission with 42.3 kHz repetition rate is observed. Figure 4(b) shows the measured pulse trains corresponding to the laser line at 1559.56 nm, where stable PQS laser pulses corresponding to the fourth pulse of the unfiltered pulses are observed. Finally, Fig. 4(c) shows the output pulse characteristics corresponding to GS pulses generated at 1862 nm. The 2-μm detector was placed at the output of the monochromator; since it detects wavelengths only up to 1.6 μm, the blue line does not match the 1862-nm laser line. The red line corresponds to the laser line at 1862 nm.
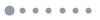
Figure 4.Profiles of the pulses filtered and unfiltered with the monochromator. (a) 1544.2 nm, (b) 1559.56 nm, and (c) 1862 nm.
Figure 5 shows the pulse trains for different pump powers, corresponding to the generated laser wavelengths at 1544.42 nm, 1559.56 nm, and 1862 nm, filtered with the monochromator. The measurements were obtained in a pump power range from 3.6 W to 7.3 W. As can be expected from typical PQS laser pulses, with the increase in pump power, the repetition rate and peak power increase as the pulse duration decreases. Figure 5(a) corresponds to the laser line generated at 1544.42 nm. A small pulse on the right corresponding to the wavelength of 1544.42 nm is observed due to the resolution of the monochromator, which does not completely block this wavelength. Figure 5(b) shows the pulse trains corresponding to the laser wavelength at 1559.56 nm. Figure 5(c) shows the GS pulses at 1862 nm. As can be expected from GS laser generation, the pulses are synchronized with the EYDCF PQS pulses, which act as a pulsed pump source to generate TDF GS pulses.
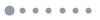
Figure 5.Measured laser pulses at different pump powers. (a) 1544.42 nm, (b) 1559.56 nm, and (c) 1862 nm.
In accordance with PQS laser operation, the four pulses in the 1.55-μm waveband observed in Fig. 4 (blue line) are separated by a time interval ; then, if the pump power is increased, the repetition rate increases as the separation between each pulse decreases in time intervals of , , and from , as reference, as shown in Fig. 6. The temporal separations , , and , obtained with minimum pump power launched, decrease to 2.96 μs, 5.8 μs, and 7.44 μs, respectively, for maximum pump power.
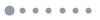
Figure 6.Separation between pulses decreases as the pump power increases.
Figure 7 shows the pulse duration and repetition rate as functions of the pump power in the range of 3.6 W to 7.3 W. As can be observed in Fig. 7(a), with the increase in pump power, the pulse duration decreases from 0.6 μs to 0.41 μs, 0.62 μs to 0.41 μs, 0.92 μs to 0.73 μs, 1.21 μs to 930 ns, 1.94 μs to 1.82 μs, 2.06 μs to 1.77 μs, 2.24 μs to 1.77 μs, 2.17 μs to 1.78 μs, and 1.42 μs to 0.94 μs for 1544.42 nm, 1545.29 nm, 1546.11 nm, 1546.98 nm, 1559.56 nm, 1560.35 nm, 1561.14 nm, 1561.89 nm, and 1862 nm, respectively. The maximal repetition rate of 75.77 kHz is obtained when the maximum pump power of 7.3 W is launched, as shown in Fig. 7(b). When the pump power increases, the pulse repetition rate increases from 43.13 kHz to 75.77 kHz. As can be seen, the repetition rate is the same for the nine generated laser wavelengths.
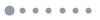
Figure 7.Characteristics of the PQS and GS laser operation. (a) Pulse duration versus pump power and (b) repetition rate versus pump power.
Figure 8 shows the blue fluorescence observed in operating the GS TDF laser. The blinking starts at a pump power of 7.5 W [Fig. 8(a)]. At this pump power level, the blinking fluorescence begins at low frequency and intensity. If the pump power increases to 7.75 W and 7.98 W, the repetition rate and intensity of the blue fluorescence blinking increase, as shown in Figs. 8(b) and 8(c), respectively. The level is responsible for the blue fluorescence observed in the operating of TDFLs [29–31]. One possible way to excite this level is as follows. In our case, using a pump in the 1550 nm range, we excite the and with a two-photon process can excite the level. Then from the , ions can be excited to the levels by photons in the 1800 nm range, and finally to the level by photons with wavelength in the 1600 nm range. This method requires high pump and signal powers. However, to understand the observed phenomenon, more detailed studies need to be conducted that go beyond the content of the present paper.
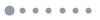
Figure 8.Blinking of TDF with different pump powers of (a) 7.5 W, (b) 7.75 W, and (c) 7.98 W.
4. CONCLUSION
In this work, we have experimentally demonstrated simultaneous PQS and GS operations of a fiber laser in the same linear cavity, where the Er/Yb PQS pulsed laser emission acts as pulsed pump power to generate GS laser pulses in the 1.8 µm wavelength range and the Tm-doped fiber is the saturable absorber for the PQS, and the gain medium for the GS laser emission. The PQS-GS fiber laser generates dual wavelengths with two peaks at corresponding to the PQS laser and a third peak around corresponding to the GS laser. The PQS spectra are two like-envelope forms that contain fourth peaks that generate fourth laser pulses. These laser pulses were filtered in order to identify each one and to know their characteristics. The -switched laser pulses serve to trigger the gain-switched laser pulses and produce a stable pulse train with a repetition rate from 43 kHz to 76 kHz and pulse duration on the order of hundreds of nanoseconds to some μs with a pump power range from 3.6 W to 7.3 W. Also, we show that with the further increasing pump power , the fiber starts to blink.
Acknowledgment
Acknowledgment. M. Durán-Sánchez was supported by the CONACyT. M. Durán-Sánchez and R. I. Álvarez-Tamayo would like to thank the Cátedras CONACyT program.
References
[1] S. D. Jackson, A. Sabella, D. G. Lancaster. Application and development of high-power and highly efficient silica-based fiber lasers operating at 2 μm. IEEE J. Sel. Top. Quantum Electron., 13, 567-572(2007).
[2] S. D. Jackson, A. Lauto. Diode pumped fiber lasers: a new clinical tool?. Lasers Surg. Med., 30, 184-190(2002).
[3] R. Szlauer, R. Götschl, A. Razmaria, L. Paras, N. T. Schmeller. Endoscopic vaporesection of the prostate using the continuous-wave 2 μm thulium laser: outcome and demonstration of the surgical technique. Eur. Urol., 55, 368-375(2009).
[4] Z. Li, A. M. Heidt, N. Simakov, Y. Jung, J. M. O. Daniel, S. U. Alam, D. J. Richardson. Diode-pumped wideband thulium-doped fiber amplifiers for optical communications in the 1800–2050 nm window. Opt. Express, 21, 26450-26455(2013).
[5] I. Mingareev, F. Weirauch, A. Olowinsky, L. Shah, P. Kadwani, M. Richardson. Welding of polymers using a 2 μm thulium fiber laser. Opt. Laser Technol., 44, 2095-2099(2012).
[6] Q. Wang, J. Geng, S. Jiang. 2-μm fiber laser sources for sensing. Opt. Eng., 53, 061609(2014).
[7] R. J. De Young, N. P. Barnes. Profiling atmospheric water vapor using a fiber laser lidar system. Appl. Opt., 49, 562-567(2010).
[8] M. Baudelet, C. C. C. Willis, L. Shah, M. Richardson. Laser-induced breakdown spectroscopy of copper with a 2 μm thulium fiber laser. Opt. Express, 18, 7905-7910(2010).
[9] D. Creeden, P. A. Ketteridge, P. A. Budni, S. D. Setzler, Y. E. Young, J. C. McCarthy, K. Zawilski, P. G. Shunemann, T. M. Pollak, E. P. Chicklis, M. Jiang. Mid-infrared ZnGeP2 parametric oscillator directly pumped by a pulsed 2 μm Tm-doped fiber laser. Opt. Lett., 33, 315-317(2008).
[10] W. Q. Yang, B. Zhang, J. Hou, R. Xiao, R. Song, Z. Liu. Gain-switched and mode-locked Tm/Ho-codoped 2 μm fiber laser for mid-IR supercontinuum generation in a Tm-doped fiber amplifier. Laser Phys. Lett., 10, 045106(2013).
[11] A. F. El-Sherif, T. A. King. High-energy, high-brightness Q-switched Tm3+-doped fiber laser using an electro-optic modulator. Opt. Commun., 218, 337-344(2003).
[12] Y. Chen, C. Zhao, S. Chen, J. Du, P. Tang, G. Jiang, H. Zhang, S. Wen, D. Tang. Large energy, wavelength widely tunable, topological insulator Q-switched erbium-doped fiber laser. IEEE J. Sel. Top. Quantum Electron., 20, 0900508(2014).
[13] R. I. Woodward, R. C. T. Howe, T. H. Runcorn, G. Hu, F. Torrisi, E. J. R. Kelleher, T. Hasan. Wideband saturable absorption in few-layer molybdenum diselenide (MoSe2) for Q-switching Yb-, Er- and Tm-doped fiber lasers. Opt. Express, 23, 20051-20061(2015).
[14] J. Li, H. Luo, B. Zhai, R. Lu, Z. Guo, H. Zhang, Y. Liu. Black phosphorus: a two-dimension saturable absorption material for mid-infrared Q-switched and mode-locked fiber lasers. Sci. Rep., 6, 30361(2016).
[15] Y. Wang, W. Huang, C. Wang, J. Guo, F. Zhang, Y. Song, Y. Ge, L. Wu, J. Liu, J. Li, H. Zhang. An all-optical, actively Q-switched fiber laser by an antimonene-based optical modulator. Laser Photon. Rev., 13, 1800313(2019).
[16] Z. Wang, H. Mu, J. Yuan, C. Zhao, Q. Bao, H. Zhang. Graphene-Bi2Te3 heterostructure as broasband saturable absorber for ultra-short pulse generation in Er-doped and Yb-doped fiber lasers. IEEE J. Sel. Top. Quantum Electron., 23, 8800105(2017).
[17] B. Wang, H. Yu, H. Zhang, C. Zhao, S. Wen, H. Zhang, J. Wang. Topological insulator simultaneously Q-switched dual-wavelength Nd:Lu2O3 laser. IEEE Photon. J., 6, 1501007(2014).
[18] R. Xiaohui, Z. Jie, Q. Xiang, Y. Liu, Z. Huang, Z. Li, Y. Ge, S. C. Dhanabalan, J. S. Ponraj, S. Wang, J. Zhong, H. Zhang. Few-layer black phosphorus nanosheets as electrocatalysts for highly efficient oxygen evolution reaction. Adv. Energy Mater., 7, 1700396(2017).
[19] M. Eichhorn, S. D. Jackson. High-pulse-energy actively Q-switched Tm3+-doped silica 2 μm fiber laser pumped at 792 nm. Opt. Lett., 32, 2780-2782(2007).
[20] M. Tao, J. Zhao, Y. Yan, Z. Wang, P. Yang, G. Feng, X. Ye. Experimental investigation of gain-switched Tm-Ho Co-doped single clad fiber lasers. Laser Phys., 23, 105101(2013).
[21] J. Swidersky, M. Maciejewska, J. Kwiatkowski, M. Mamajek. An all-fiber, resonantly pumped, gain-switched, 2 μm Tm-doped silica fiber laser. Laser Phys. Lett., 10, 015107(2013).
[22] P. Grzes, J. Swiderski. Gain-switched 2-μm fiber laser system providing kilowatt peak-power mode-locked resembling pulses and its application to supercontinuum generation in fluoride fibers. IEEE Photon. J., 10, 1500408(2018).
[23] M. Jiang, P. Tayebati. Stable 10 ns, kilowatt peak-power pulse generation from a gain-switched Tm-doped fiber laser. Opt. Lett., 32, 1797-1799(2007).
[24] Y. Tang, F. Li, J. Xu. High peak-power gain-switched Tm3+-doped fiber laser. IEEE Photon. Technol. Lett., 23, 893-895(2011).
[25] N. Simakov, A. Hemming, S. Bennetts, J. Haub. Efficient, polarised, gain-switched operation of a Tm-doped fibre laser. Opt. Express, 19, 14949-14954(2011).
[26] J. Yang, Y. Tang, J. Xu. Development and applications of gain-switched fiber lasers. Photon. Res., 1, 52-57(2013).
[27] B. Ibarra-Escamilla, M. Durán-Sánchez, R. I. Álvarez-Tamayo, B. Posada-Ramírez, P. Prieto-Cortes, J. Alaniz-Baylón, H. Santiago-Hernandez, M. Bello-Jiménez, E. A. Kuzin. All-fiber laser with simultaneous Tm3+ passive Q-switched and Ho3+ gain-switched operation. Opt. Lett., 43, 3377-3380(2018).
[28] B. Posada-Ramírez, M. Durán-Sánchez, R. I. Álvarez-Tamayo, J. Alaniz-Baylón, B. Ibarra-Escamilla, E. A. Kuzin. All-fiber multi-wavelength passive Q-switched Er/Yb fiber laser based on a Tm-doped fiber saturable absorber. Laser Phys., 27, 035103(2017).
[29] S. D. Jackson, T. A. King. Theorical modeling of Tm-doped silica fiber lasers. J. Lightwave Technol., 17, 948-956(1999).
[30] P. F. Moulton, G. A. Rines, E. V. Slobodtchikov, K. F. Wall, G. Frith, B. Samson, A. L. G. Carter. Tm-doped fiber lasers: fundamentals and power scaling. IEEE J. Sel. Top. Quantum Electron., 15, 85-92(2009).
[31] P. Peterka, B. Faure, W. Blanc, M. Karasek, B. Dussardier. Theoretical modeling of S-band thulium-doped silica fibre amplifiers. Opt. Quantum Electron., 36, 201-212(2004).