Abstract
We demonstrate a simple technique to filter out the continuum background in filament-induced remote breakdown spectroscopy. By inserting a polarizer before the detector, the continuum background was reduced by more than 42% in filament-induced breakdown spectroscopy at a distance of 3.8 m, while the fluorescence intensity of aluminum atomic lines remains constant. Supercontinuum through self-phase modulation during filamentation mainly contributes to the continuum background. The polarization-gated technique provides a simple way to remove the continuum background in filament-induced remote breakdown spectroscopy.During its propagation in air, an intense femtosecond laser pulse experiences Kerr self-focusing and plasma defocusing, resulting in the formation of self-guided plasma channels named filaments, inside which laser peak intensity is clamped to around in air[1]. Filament-induced breakdown spectroscopy (FIBS) that combines filamentation with laser-induced breakdown spectroscopy (LIBS) is a versatile tool for elemental and molecular analysis. Because of the self-focusing effect, FIBS is capable of delivering high intensity at long distances, which makes FIBS a superior technique for remote sensing of biologic materials[2,3], aerosols[4], and metals[5]. However, other nonlinear processes, such as self-phase modulation (SPM) and self-stepping, unavoidably occur during long distance filamentation, which results in strong spectral broadening, i.e., supercontinuum generation. The broadened spectra can span from the ultraviolet to the mid-infrared[6]. Thus, it is difficult to distinguish useful fluorescence signals from the backscattered supercontinuum in remote sensing application of FIBS.
The most well-known technique to reduce the effect of supercontinuum is time-resolved LIBS (TR-LIBS). In this method, an intensified CCD (ICCD) camera is used to suppress the supercontinuum by selecting an appropriate gate width and delay time, because the lifetime of the supercontinuum is much shorter than the lifetime of discrete fluorescence[1,2,7]. However, there are several limits on this method, e.g., the ICCD is much more expensive than the photomultiplier tube (PMT), CCD, and other detectors. The detection gate width and delay of the ICCD are very sensitive to the target samples, which are mostly unknown parameters before experiments.
Recently, a novel technique was demonstrated by Zhao et al.[8] to reduce the plasma continuum radiation in dual-pulse femtosecond LIBS experiments, which was referred to as polarization-resolved LIBS. By inserting a polarizer before the detector and tuning the transmission angle of the polarizer, the plasma continuum background was significantly filtered out, while the atomic emission spectral lines retain their intensity. Since then, a few works have been carried out to understand the polarization property of the continuum background; however, the underlying mechanism is still under debate[9–14]. It should be noted that the aforementioned works were carried out with either nanosecond lasers or femtosecond lasers with laser power much weaker than critical power for filamentation. Note that the critical power of self-focusing for filamentation in air was measured to be about 10 GW for a 42 fs pulse[15]. Applying a laser filament into breakdown spectroscopy will not only improve the intensity stability of laser-induced fluorescence because of laser intensity clamping during filamentation[16], but it also has the capability for remote applications[2,6,7,17]. The techniques to remove the filament-induced supercontinuum generation from breakdown fluorescence are highly needed for remote sensing applications.
Sign up for Chinese Optics Letters TOC. Get the latest issue of Chinese Optics Letters delivered right to you!Sign up now
In this Letter, we apply the polarization-resolved technique to remote FIBS, which we refer to as polarization-gated filament-induced remote breakdown spectroscopy. By simply placing a polarizer before the detector, the filament-induced supercontinuum background was successfully filtered out in the filament-induced breakdown spectra at a distance of 3.8 m. The validity of this technique has been further proved by rotating the laser polarization by 95° at the same distance. The origin of the continuum background is also discussed and identified.
The schematic of our experimental setup is shown in Fig. 1. A Ti:sapphire laser was used in our experiments to generate laser pulses with a repetition rate of 1 kHz. Through a telescope based remote focusing system, which consists of a pair of lenses, [, diameter , fused silica with anti-reflection (AR) coating at 800 nm] and (, diameter , N-BK7 with AR coating at 800 nm). By changing the distance between and , the location where the filament was formed could be tuned and well controlled. A half-wave plate was inserted before to control the polarization state of the laser pulses. An Al plate was used as the sample, which was mounted on a two-dimensional rotation stage with a rotating rate of 30° per second. The laser filament was formed several meters away to ablate Al alloy in air, and the laser filament was fired perpendicularly to the sample surface (Fig. 1). The backward filament-induced breakdown fluorescence signals were collected by an Al-coated concave mirror (, diameter ) and then coupled into a fiber to the spectrometer (Princeton Instruments, SP-2560) for spectral analysis. The FIBS signals were dispersed by a grating bladed at 500 nm and recorded by a CCD camera (Princeton Instruments, PIXIS: 1024B eXcelon). The CCD exposure time was 10 ms, and all the spectra obtained in the following were the sum of 100 laser shots through a 10 times accumulation. The entrance slit of the spectrometer was 20 μm. A short-pass filter (Thorlabs, FESH0750, cut-off longer wavelength: 750 nm) was mounted before the fiber head to protect the spectrometer and CCD from the strong exciting laser pulses. A polarizer (Thorlabs, LPNIRE100-B) was also inserted before the fiber head to allow different polarization components to pass through.
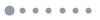
Figure 1.Schematic of the experimental setup: HWP, half-wave plate at 800 nm; , plano-concave lens ();, plano-convex lens (); , concave mirror with Al coating ().
The spectral window around the two peaks of Al atomic lines (394 nm and 396 nm) was chosen in the following measurements and analysis. Figure 2(a) shows the filament-induced breakdown spectra of Al under different polarizer angles of , 15°, 45°, 60°, and 105°. Note that corresponds to the polarization plane of the incident laser beam. The distance between and was 14.2 cm, and the samples were 3.80 m away from . The pulse duration was 30 fs (full width at half-maximum measured at the output port of the laser), and the laser pulse energy before was 6.22 mJ; the laser power was about 200 GW, which was well above critical power in air[15]. It is clearly seen that the intensity of the background is significantly reduced by about 42% (calculated at 420.171 nm where no special line is observed) when the detective angle of the polarizer is tuned from to 60°, indicating that the background of FIBS is highly polarized. The intensity of the Al atomic line (for instance, Al I 396.152 nm) and the background of this line (referred to as background in the following discussion) change simultaneously, while we alter the polarizer angle, suggesting the ‘real’ intensity of the Al atomic line may be independent of the polarizer angle.
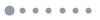
Figure 2.(a) Filament-induced breakdown spectra of Al under different polarizer angles of , 15°, 45°, 60°, and 105°. was corresponding to the polarization plane of the laser beam. The energy before was 6.22 mJ, and the pulse duration was 30 fs. (b) Integration range used to calculate the integral intensity of Al I 396.152 nm and its background.
To verify our assumption, we integrate the spectral intensity from 395.846 to 399.273 nm to get the integral intensity of Al I 396.152 nm. The integration range is shown in Fig. 2(b). The interpolation method was adapted to obtain the baseline of the spectra, and then the background intensity of Al I 396.152 nm was calculated by the integration of the spectral intensity of the same integration range. Then, we subtract the background of Al I 396.152 nm from its original signal, and the residual is referred to as relative intensity in the following discussion. The relative intensity as a function of the detective angle of the polarizer is shown in Fig. 3 together with the background and original spectral intensities. The black and blue lines in Fig. 3 are the fitting curves through a Malus function [] to the intensities. The red dot line shows the polarization of the laser, which was measured before . As shown in Fig. 3, the continuum background experiences the minimum at the angle around 65°, which corresponds to the direction perpendicular to laser polarization direction. The intensity of the continuum background signal nicely follows the polarization of the laser pulse, while the relative intensity, which indicates the fluorescence intensity of the Al atomic line, remains almost at a constant value. The different behavior may indicate the different origins. The polarization-gated technique provides a simple method to remove the continuum background in filament-induced remote breakdown spectroscopy.
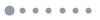
Figure 3.Comparison of polarization dependence of relative intensity, original intensity, and background intensity of the Al atomic line (Al I 396.152 nm) together with the polarization of the incident laser beam.
To further approve the effectiveness of polarization-gated FIBS, the polarization direction of the incident laser beam was rotated by 95° with a half-wave plate inserted before . The polarizer angle in the transmission direction of laser polarization was 70° after rotation. With the same filament projecting and signal detecting configuration, similar measurement and analysis as Fig. 3 were made at a distance of 3.8 m. The results are shown in Fig. 4. At the polarizer angle of , a maximum of the continuum background was observed, which is consistent with the direction of laser polarization. Again, the background signal nicely follows the polarization of the incident laser pulse, which confirms again its polarization property. The relative intensity of the Al atomic line remains almost constant compared with the background signal, which confirms that the ‘real’ atomic line of Al has no polarization property.
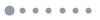
Figure 4.Spectral intensity of the Al atomic line (original data), background, and relative intensity as a function of the polarizer angle after inserting a half-wave plate before the telescope system.
Considering the origin of the polarization-dependent continuum background, there are two different kinds of contributions that should be considered in FIBS, i.e., supercontinuum from the nonlinear SPM process[7] and plasma continuum emission from the radiative recombination of electrons and ions and inverse bremsstrahlung[8]. According to Refs. [12,13], the polarization of plasma continuum emission is independent of the polarization state of the incident laser beam. The intensity of the plasma continuum is always stronger along the s-polarization direction. On the contrary, the polarization of the continuum background is strongly dependent on the polarization of the incident laser in this work. Since the polarization of the filament-induced supercontinuum through SPM is polarized in the same direction as the incident laser pulse in isotropic media[18], the SPM supercontinuum should be dominate the continuum background in filament-induced breakdown spectra, which is consistent with other remote sensing experiments using filaments[7,19]. Moreover, due to a lower temperature of filament-induced plasma compared with nanosecond LIBS (ns-LIBS), the continuum emission from plasma in FIBS is much weaker compared with ns-LIBS[19,20].
Recently, it has been reported that a circularly polarized femtosecond laser pulse can produce a higher intensity inside the filament core[21], which may be more useful for remote sensing. However, the polarization-gated technique in this work may be much less useful in FIBS experiments with circularly polarized laser than with linearly polarized laser, because the continuum background induced by a circularly polarized filamenting pulse cannot be filtered out from the collected signal.
In summary, we demonstrate that the continuum background in filament-induced remote breakdown spectroscopy could be significantly filtered out by inserting a polarizer in front of the detector while the atomic lines of Al remain at a constant intensity. The continuum background in FIBS, which was identified as the supercontinuum generation through SPM during filamentation, is polarized in the plane of the incident laser polarization. The polarization-gated technique is very simple and will find applications in filament-induced remote breakdown spectroscopy.
References
[1] S. L. Chin, S. A. Hosseini, W. Liu, Q. Luo, F. Theberge, N. Akozbek, A. Becker, V. P. Kandidov, O. G. Kosareva, H. Schroeder. Can. J. Phys., 83, 863(2005).
[2] H. L. Xu, W. Liu, S. L. Chin. Opt. Lett., 31, 1540(2006).
[3] J. F. Daigle, Y. Kamali, G. Roy, S. Chin. Appl. Phys. B-Lasers Opt., 93, 759(2008).
[4] J. F. Daigle, G. Mejean, W. Liu, F. Theberge, H. L. Xu, Y. Kamali, J. Bernhardt, A. Azarm, Q. Sun, P. Mathieu, G. Roy, J. R. Simard, S. L. Chin. Appl. Phys. B-Lasers Opt., 87, 749(2007).
[5] B. Zeng, T. J. Wang, S. Hosseini, Y. Cheng, Z. Z. Xu, W. W. Liu, S. L. Chin. J. Opt. Soc. Am. B-Opt. Phys., 29, 3226(2012).
[6] S. L. Chin, H. L. Xu, Q. Luo, F. Theberge, W. Liu, J. F. Daigle, Y. Kamali, P. T. Simard, J. Bernhardt, S. A. Hosseini, M. Sharifi, G. Mejean, A. Azarm, C. Marceau, O. Kosareva, V. P. Kandidov, N. Akozbek, A. Becker, G. Roy, P. Mathieu, J. R. Simard, M. Chateauneuf, J. Dubois. Appl. Phys. B-Lasers Opt., 95, 1(2009).
[7] H. L. Xu, G. Mejean, W. Liu, Y. Kamali, J. F. Daigle, A. Azarm, P. T. Simard, P. Mathieu, G. Roy, J. R. Simard, S. L. Chin. Appl. Phys. B-Lasers Opt., 87, 151(2007).
[8] Y. B. Zhao, S. Singha, Y. M. Liu, R. J. Gordon. Opt. Lett., 34, 494(2009).
[9] G. A. Wubetu, H. Fiedorowicz, J. T. Costello, T. J. Kelly. Phys. Plasmas, 24, 013105(2017).
[10] Y. M. Liu, J. S. Penczak, R. J. Gordon. Opt. Lett., 35, 112(2010).
[11] J. S. Penczak, Y. M. Liu, R. J. Gordon. Spectrochim. Acta Part B-At. Spectros., 66, 186(2011).
[12] J. S. Penczak, Y. Liu, R. D. Schaller, D. H. Rich, R. J. Gordon. Spectrochim. Acta Part B-At. Spectros., 74–75, 3(2012).
[13] A. E. Majd, A. S. Arabanian, R. Massudi. Opt. Lasers Eng., 48, 750(2010).
[14] M. E. Asgill, H. Y. Moon, N. Omenetto, D. W. Hahn. Spectrochim. Acta Part B-At. Spectros., 65, 1033(2010).
[15] W. Liu, S. L. Chin. Opt. Express, 13, 5750(2005).
[16] N. Chen, Y. X. Liu, T. J. Wang, Z. B. Zhu, H. Y. Sun, X. N. Yan, Y. H. Xia, J. H. Xia, J. Du, J. S. Liu, Y. X. Leng. Chin. Opt. Lett., 15, 061902(2017).
[17] H. L. Xu, Y. Cheng, S. L. Chin, H. B. Sun. Laser Photon. Rev., 9, 275(2015).
[18] R. R. Alfano, S. L. Shapiro. Phys. Rev. Lett., 24, 592(1970).
[19] H. L. Xu, J. Bernhardt, P. Mathieu, G. Roy, S. L. Chin. J. Appl. Phys., 101, 6(2007).
[20] T. A. Labutin, V. N. Lednev, A. A. Ilyin, A. M. Popov. J. Anal. At. Spectrom., 31, 90(2016).
[21] C. Liu, H. Zang, H. Li, Y. Yu, H. Xu. Chin. Opt. Lett., 15, 120201(2017).