Zong-Lu Che, Chang-Cun Yan, Xue-Dong Wang, Liang-Sheng Liao, "Organic near-infrared optoelectronic materials and devices: an overview," Adv. Photon. 6, 014001 (2024)

Search by keywords or author
- Advanced Photonics
- Vol. 6, Issue 1, 014001 (2024)
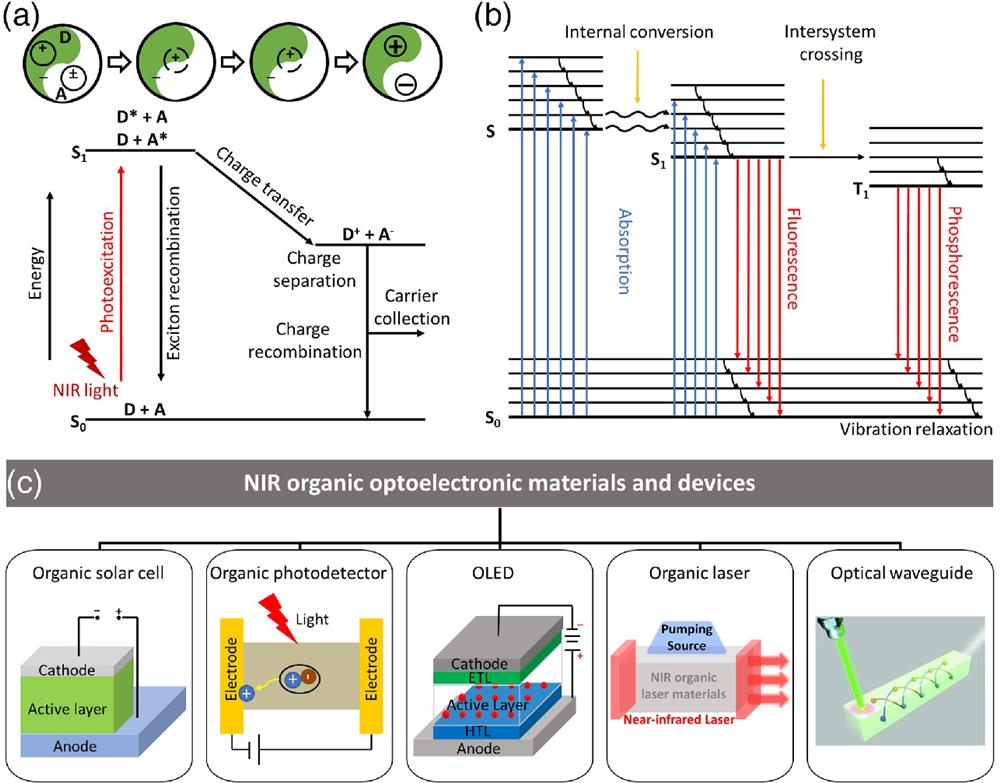
Fig. 1. (a) Mechanism of photogenerated charge transfer. (b) Mechanisms of fluorescence and phosphorescence. (c) Main contents of this review.
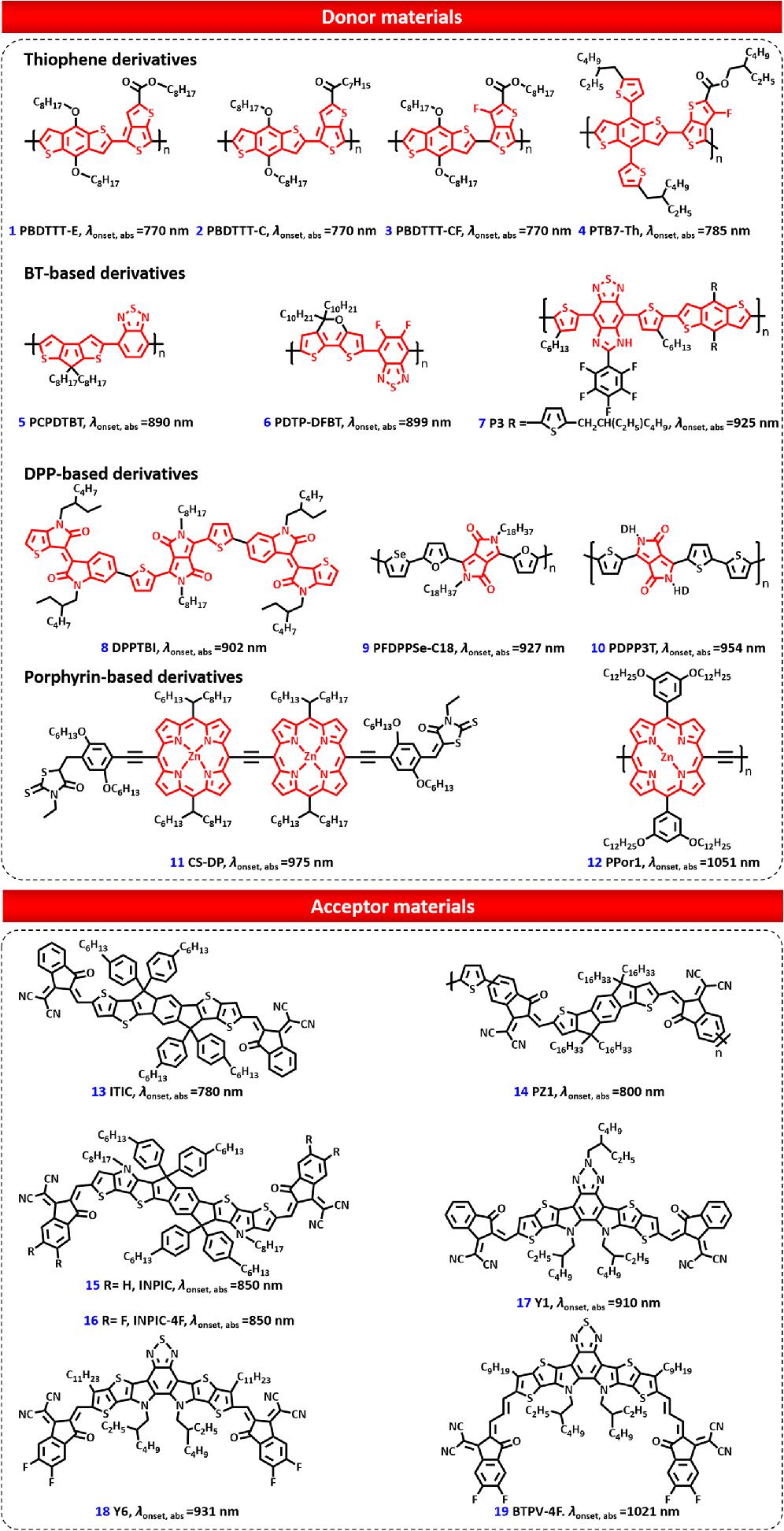
Fig. 2. Typical donor and acceptor materials for NIR OSCs.
![(a) Device structure combining ST-OSC and 3-DM. (b) Transmission spectra of ST-OSC with different active layers. (c) Transmission spectra of different active layers added with 3-DMs. (d) Photographs of the ternary ST-OSC and glass coating. (e) Schematic diagram of the device structure of the tandem solar cell. (f) J−V curve of Tandem 1 and Tandem 2. (g) Absorption spectra of ITIC in solution (dichloromethane) and films. (h) J−V curves of PSCs with different acceptors. (i) Schematic diagrams of active layers. (j) Optical photograph of a demo [left: glass/ITO/ZnO/PBDB-T:PTAA:Y1 (6:1:9)/MoO3/Au/Ag; right: PET/Ag mesh/PEDOT:PSS PH1000/ZnO/PBDB-T:PTAA:Y1 (6:1:9)/MoO3/Au/Ag]. [(a), (b), (c), (d) Reproduced with permission,72" target="_self" style="display: inline;">72 © 2019 WILEY-VCH. (e), (f) Reproduced with permission,65" target="_self" style="display: inline;">65 © 2013 Macmillan Publishers Limited. (g), (h) Reproduced with permission,73" target="_self" style="display: inline;">73 © 2015 WILEY-VCH. (i), (j) Reproduced with permission,74" target="_self" style="display: inline;">74 © 2020 WILEY-VCH.]](/Images/icon/loading.gif)
Fig. 3. (a) Device structure combining ST-OSC and 3-DM. (b) Transmission spectra of ST-OSC with different active layers. (c) Transmission spectra of different active layers added with 3-DMs. (d) Photographs of the ternary ST-OSC and glass coating. (e) Schematic diagram of the device structure of the tandem solar cell. (f) curve of Tandem 1 and Tandem 2. (g) Absorption spectra of ITIC in solution (dichloromethane) and films. (h) curves of PSCs with different acceptors. (i) Schematic diagrams of active layers. (j) Optical photograph of a demo [left: glass/ITO/ZnO/PBDB-T:PTAA:Y1 (6:1:9)/MoO3/Au/Ag; right: PET/Ag mesh/PEDOT:PSS PH1000/ZnO/PBDB-T:PTAA:Y1 (6:1:9)/MoO3/Au/Ag]. [(a), (b), (c), (d) Reproduced with permission,72 © 2019 WILEY-VCH. (e), (f) Reproduced with permission,65 © 2013 Macmillan Publishers Limited. (g), (h) Reproduced with permission,73 © 2015 WILEY-VCH. (i), (j) Reproduced with permission,74 © 2020 WILEY-VCH.]
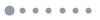
Fig. 4. Representative small molecule and polymer materials for NIR OPDs.
![(a) Schematic of the device structure of ultraflexible NIR OPDs. (b) Transmittance of parylene on different substances. (c) Photograph of fingerprint-conformal NIR OPDs, and the inset indicates the position of the skin-conformal NIR OPDs on the finger. (d) Device structure of the NIR OPDs. (e) Absorption spectra of PTB7-Th (red), CO1-4Cl (blue), and their BHJ blend (purple) in thin films. (f) Working principle of NIR photoplethysmography. [(a)–(c) Reproduced with permission,100" target="_self" style="display: inline;">100 © 2018 WILEY-VCH. (d)–(f) Reproduced with permission,107" target="_self" style="display: inline;">107 © 2019 WILEY-VCH.]](/Images/icon/loading.gif)
Fig. 5. (a) Schematic of the device structure of ultraflexible NIR OPDs. (b) Transmittance of parylene on different substances. (c) Photograph of fingerprint-conformal NIR OPDs, and the inset indicates the position of the skin-conformal NIR OPDs on the finger. (d) Device structure of the NIR OPDs. (e) Absorption spectra of PTB7-Th (red), CO1-4Cl (blue), and their BHJ blend (purple) in thin films. (f) Working principle of NIR photoplethysmography. [(a)–(c) Reproduced with permission,100 © 2018 WILEY-VCH. (d)–(f) Reproduced with permission,107 © 2019 WILEY-VCH.]
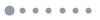
Fig. 6. (a) Mechanism of emitting light of three generations of OLEDs. (b)–(d) Representative NIR materials for each of the three generations of OLEDs.
![(a) OLED EQE of the materials mentioned in the article. (b) Crystal structure and intermolecular interaction of TBSMCN. (c) EL spectra of devices with different structures of TBSMCN. (d) EQE of devices with different structures of TBSMCN at different current densities. [(b)–(d) Reproduced with permission,117" target="_self" style="display: inline;">117 © 2022 WILEY-VCH.]](/Images/icon/loading.gif)
Fig. 7. (a) OLED EQE of the materials mentioned in the article. (b) Crystal structure and intermolecular interaction of TBSMCN. (c) EL spectra of devices with different structures of TBSMCN. (d) EQE of devices with different structures of TBSMCN at different current densities. [(b)–(d) Reproduced with permission,117 © 2022 WILEY-VCH.]
![(a) Molecular structures of LDS 798. (b) Schematic illustration of the formation of LDS 798@rho-ZMOF microcrystals via an ion exchange process. (c) Room-temperature PL spectra of an individual LDS 798@rho-ZMOF microcrystal under different pump densities at 532 nm. (d1)–(d3) The molecular structures of compounds 64, 65, and 66. (e) PL spectra of compound 64 in doped films at different pumping energies. (f) Laser emission spectra of compound 64 in doped films of different concentrations. (g1) Schematic diagram of the dye-doped film. (g2) The lasing emission spectra from the active waveguide with the FP cavity at increasing excitation levels near the threshold. (h) Molecular structure of ADH. (i) Calculated relative energies (kcal mol−1) of the TA and TB forms of NDH. (j) Laser emission spectra of ADH in PS spheres. [(b), (c) Reproduced with permission,162" target="_self" style="display: inline;">162 © 2018 American Chemical Society (ACS). (e), (f) Reproduced with permission,161" target="_self" style="display: inline;">161 © 2018 ACS. (g2) Reproduced with permission,158" target="_self" style="display: inline;">158 © 2008 American Institute of Physics. (i), (j) Reproduced with permission,159" target="_self" style="display: inline;">159 © 2020 WILEY-VCH.]](/Images/icon/loading.gif)
Fig. 8. (a) Molecular structures of LDS 798. (b) Schematic illustration of the formation of LDS 798@rho-ZMOF microcrystals via an ion exchange process. (c) Room-temperature PL spectra of an individual LDS 798@rho-ZMOF microcrystal under different pump densities at 532 nm. (d1)–(d3) The molecular structures of compounds 64, 65, and 66. (e) PL spectra of compound 64 in doped films at different pumping energies. (f) Laser emission spectra of compound 64 in doped films of different concentrations. (g1) Schematic diagram of the dye-doped film. (g2) The lasing emission spectra from the active waveguide with the FP cavity at increasing excitation levels near the threshold. (h) Molecular structure of ADH. (i) Calculated relative energies ( ) of the TA and TB forms of NDH. (j) Laser emission spectra of ADH in PS spheres. [(b), (c) Reproduced with permission,162 © 2018 American Chemical Society (ACS). (e), (f) Reproduced with permission,161 © 2018 ACS. (g2) Reproduced with permission,158 © 2008 American Institute of Physics. (i), (j) Reproduced with permission,159 © 2020 WILEY-VCH.]
![(a) Molecular structures of 67, 68, 69. (b1), (b2) PL spectra of compounds 67 and 68. (c) Images of 1R, 1O, heated 1O, and the heated phase transition of 1O. (d) PL spectra of 1R. (e) Chemical structures of representative materials. (f) PL spectra of 11.5 μm long single nanowires excited by different pump energies. Inset: SEM image of organic nanowires. (g) Schematic of one nanowire on a glass substrate pumped by 532 nm laser excitation. (h) Fluorescence microscopy image of these as-prepared DMHC organic nanowire arrays. (i) PL spectra based on a single nanowire with a length of 10 μm excited at different energies at room temperature. (j) Multimode laser spectra of the selected DP-DHAQ microplate when excited by a pulsed laser (532 nm). Inset: fluorescence microscopy image of the selected microplate above the lasing threshold. [(b1), (b2) Reproduced with permission,163" target="_self" style="display: inline;">163 © 2015 WILEY-VCH. (c), (d) Reproduced with permission,164" target="_self" style="display: inline;">164 © 2016 WILEY-VCH. (f) Reproduced with permission,169" target="_self" style="display: inline;">169 © 2021 WILEY-VCH. (g)–(i) Reproduced with permission,168" target="_self" style="display: inline;">168 © 2020 Elsevier Inc. (j) Reproduced with permission,171" target="_self" style="display: inline;">171 © 2022 WILEY-VCH.]](/Images/icon/loading.gif)
Fig. 9. (a) Molecular structures of 67, 68, 69. (b1), (b2) PL spectra of compounds 67 and 68. (c) Images of 1R, 1O, heated 1O, and the heated phase transition of 1O. (d) PL spectra of 1R. (e) Chemical structures of representative materials. (f) PL spectra of long single nanowires excited by different pump energies. Inset: SEM image of organic nanowires. (g) Schematic of one nanowire on a glass substrate pumped by 532 nm laser excitation. (h) Fluorescence microscopy image of these as-prepared DMHC organic nanowire arrays. (i) PL spectra based on a single nanowire with a length of excited at different energies at room temperature. (j) Multimode laser spectra of the selected DP-DHAQ microplate when excited by a pulsed laser (532 nm). Inset: fluorescence microscopy image of the selected microplate above the lasing threshold. [(b1), (b2) Reproduced with permission,163 © 2015 WILEY-VCH. (c), (d) Reproduced with permission,164 © 2016 WILEY-VCH. (f) Reproduced with permission,169 © 2021 WILEY-VCH. (g)–(i) Reproduced with permission,168 © 2020 Elsevier Inc. (j) Reproduced with permission,171 © 2022 WILEY-VCH.]
![(a) Fluorescence microscopy images of Pb-Bpeb. Inset: molecular structure of Pb-Bpeb. (b) 1D crystal by exciting the individual crystals at different positions with a laser beam (λ=405 nm). (c) Intensity ratio Itip/Ibody against the distance. (d) Molecular structure of DHNBP and the diagram of the primary branched microwire. (e) Schematic illustration of the experimental setup used for optical waveguide measurement. (f) FM images obtained from an individual TP-F4TCNQ microwire by excitation with a laser beam (λ=375 nm) at different positions with a scale bar of 50 μm. (g) Corresponding spatially resolved PL spectra in (f) with different separation distances. Inset: ratios of the intensity Itip/Ibody against the distance d. (h) Molecular structure of DCA and DPI, schematic representation of the waveguide with relative dimensions, and the procedure for manual loading with PDI crystals. [(a)–(c) Reproduced with permission,177" target="_self" style="display: inline;">177 © 2021 ACS. (e)–(g) Reproduced with permission,178" target="_self" style="display: inline;">178 © 2022 WILEY-VCH.]](/Images/icon/loading.gif)
Fig. 10. (a) Fluorescence microscopy images of Pb-Bpeb. Inset: molecular structure of Pb-Bpeb. (b) 1D crystal by exciting the individual crystals at different positions with a laser beam ( ). (c) Intensity ratio against the distance. (d) Molecular structure of DHNBP and the diagram of the primary branched microwire. (e) Schematic illustration of the experimental setup used for optical waveguide measurement. (f) FM images obtained from an individual microwire by excitation with a laser beam ( ) at different positions with a scale bar of . (g) Corresponding spatially resolved PL spectra in (f) with different separation distances. Inset: ratios of the intensity against the distance . (h) Molecular structure of DCA and DPI, schematic representation of the waveguide with relative dimensions, and the procedure for manual loading with PDI crystals. [(a)–(c) Reproduced with permission,177 © 2021 ACS. (e)–(g) Reproduced with permission,178 © 2022 WILEY-VCH.]
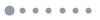
Fig. 11. Present challenges in organic NIR optoelectronic materials.
|
Table 1. Optoelectronic and photovoltaic properties of representative NIR donor materials in OSCs.
|
Table 2. Optoelectronic and photovoltaic properties of representative NIR acceptor materials in OSCs.
|
Table 3. Optoelectronic properties for polymer and small molecule absorption materials and devices incorporating them.
|
Table 4. Optoelectronic properties for representative fluorescent materials and corresponding devices.
|
Table 5. Optoelectronic properties for representative phosphorescent materials and corresponding devices.
|
Table 6. Optoelectronic properties for TADF materials and corresponding devices.
|
Table 7. Properties of NIR OSSLs.
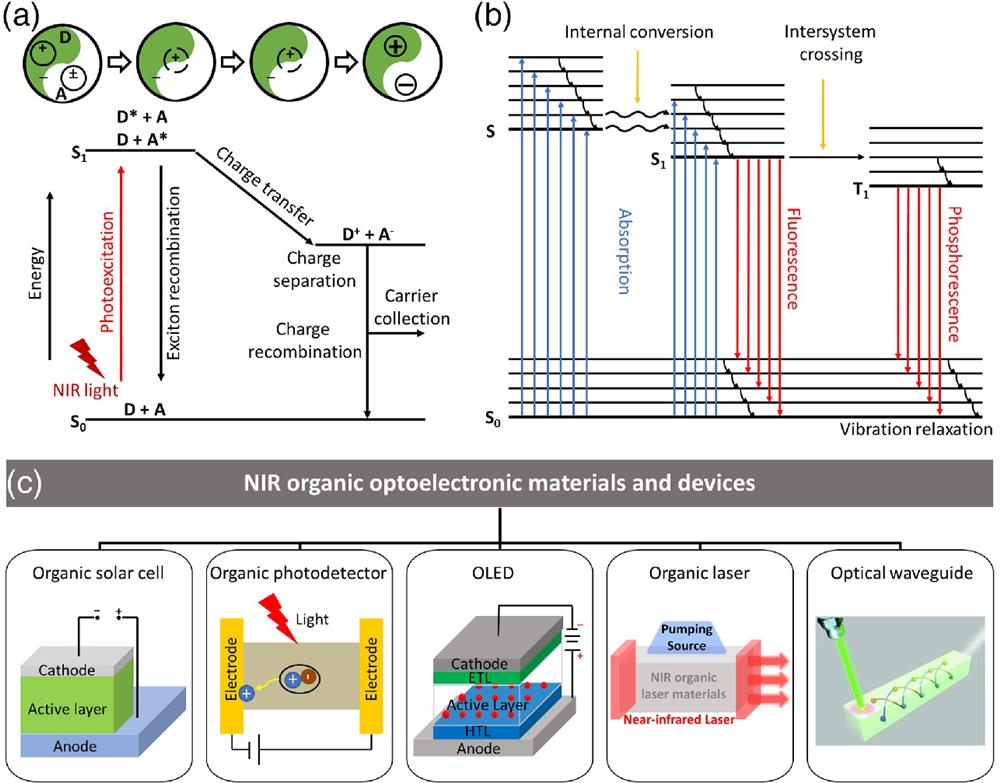
Set citation alerts for the article
Please enter your email address