Abstract
Long-term optical data storage (ODS) technology is essential to break the bottleneck of high energy consumption for information storage in the current era of big data. Here, ODS with an ultralong lifetime of 2×107 years is attained with single ultrafast laser pulse induced reduction of Eu3+ ions and tailoring of optical properties inside the Eu-doped aluminosilicate glasses. We demonstrate that the induced local modifications in the glass can stand against the temperature of up to 970 K and strong ultraviolet light irradiation with the power density of 100 kW/cm2. Furthermore, the active ions of Eu2+ exhibit strong and broadband emission with the full width at half maximum reaching 190 nm, and the photoluminescence (PL) is flexibly tunable in the whole visible region by regulating the alkaline earth metal ions in the glasses. The developed technology and materials will be of great significance in photonic applications such as long-term ODS.Long-term optical data storage (ODS) technology is essential to break the bottleneck of high energy consumption for information storage in the current era of big data. Here, ODS with an ultralong lifetime of 2×107 years is attained with single ultrafast laser pulse induced reduction of Eu3+ ions and tailoring of optical properties inside the Eu-doped aluminosilicate glasses. We demonstrate that the induced local modifications in the glass can stand against the temperature of up to 970 K and strong ultraviolet light irradiation with the power density of 100 kW/cm2. Furthermore, the active ions of Eu2+ exhibit strong and broadband emission with the full width at half maximum reaching 190 nm, and the photoluminescence (PL) is flexibly tunable in the whole visible region by regulating the alkaline earth metal ions in the glasses. The developed technology and materials will be of great significance in photonic applications such as long-term ODS.Introduction
In the development history of human society, data storage plays an indispensable and pivotal role, it has significantly boosted spacious domains from social science to industrial production. With the advent of the Internet of Things and artificial intelligence, the long lifetime and large capacity of information storage are in increasingly high demand. Accordingly, optical data storage (ODS) technique provides a highly fascinating alternative to conventional storage techniques (semiconductor or magnetic-based storage) with lower energy consumption, larger capacity, higher efficiency, and longer lifetime1-5. However, accounting for 80% of the total stored data generated by the government, data disaster recovery centers, and archives are not frequently accessed but still need to be available over long periods in an easy access method. The typical lifetime of mature ODS is hundreds of years that is still far from meeting the requirements of next-generation information storage6-8. Although the ODS based on ultrafast laser induced volume periodic structures has an ultralong lifetime, multipulse irradiation with the pulse energy up to micro-joule is necessary for writing, which will slow down the writing speed and increase the power consumption9. Developing long-term stable ODS based on single pulse writing process can not only notably reduce the energy dissipation associated with frequent data migration but also increase the efficiency of data writing.
Tailoring of optical properties of active centers or dopants in solid matrix has extensively boosted the applications of glasses and crystals in optical communication, information storage, photonic device, and display2, 10-13. Typically, the spectroscopic features of the active centers or dopants are highly dependent on the local structures of amorphous glass or crystal field and generally tuned via chemical component modulation and homogeneous heat treatment of the matrix14-17. Recently, ultrafast laser has also been established to be an effective tool for tailoring optical properties of transparent solids and hence implementing new functionalities with three-dimensional (3D) structuring capability18-23. For example, modifying the local structures and valence of active ions in transparent matrix endows writing information in the local volume by distinct photoluminescence (PL) emission properties that enable large capacity ODS with a high signal-to-noise ratio24-27. However, the PL is generally induced by multipulse laser irradiation, which limits the writing speed of ODS. Besides, an obvious decrease in the PL intensity of active ions is usually observable at high temperatures, which is detrimental for long-term device operation28-30. Hence, the lifetime of ODS based on the distinct PL is limited to only a few hundred years or even several decades1, 31. It is a challenge to realize ODS that not only possesses a facile method and fast writing speed but also possesses an ultralong lifetime and excellent stability.
In this work, we report long-term and high writing speed ODS by tailoring the valence state of Eu3+ ions inside glass with single laser pulse. We demonstrate that the lifetime of stored information is as long as 2×107 years and the written local modifications can stand against the temperature of up to 970 K and intense ultraviolet irradiation (100 kW/cm2). Furthermore, broadband emission of the active Eu2+ ions is observed with the full width at half maximum (FWHM) reaching 190 nm and the PL can be tuned in the whole visible region.
Results and discussion
Ordinarily, luminescent materials, a kind of widely employed ODS medium, are composed of a reasonable combination of certain active dopants with an inert matrix. Divalent europium cation (Eu2+) is one of the extensively applied activators due to unequaled 4f-5d transition32-34. The emission spectrum of Eu2+ is largely dependent on the chemical compositions and structures of hosts as the 5d orbital is sensitive to the surrounding environment. Besides, aluminosilicate glass is considered as an outstanding category of host matrix due to the excellent stability and accessible synthesis method with relatively low cost of raw materials. Eu-doped earth-abundant aluminosilicate glass represents preeminent luminescence characteristics that can meet distinct optoelectronic applications. Therefore, Eu2O3 doped calcium-silicon-aluminum (Eu: CSA) glass is employed to serve as ODS matrix, among which intermediate elements including silicium and aluminum act as glass former, whereas the alkaline earth metal element (calcium) serves as glass modifier. Ultrafast laser is used to tailor PL properties of Eu: CSA glass to enable data writing. During laser-matter interaction, three-photon absorption occurs in the laser modified area, and active electrons and holes can be created in glass through multi-photon ionization process. When the electrons are trapped by Eu3+, permanent photoreduction of Eu3+ will be achieved to generate Eu2+, and different luminescence characters will be attained.
Figure 1(a) shows the PL spectra from Eu3+ in initial Eu: CSA glass before tailoring luminescence by femtosecond (fs) laser. A series of characteristic emission lines between 570 and 750 nm attributed to the
transition appear8, 35. The target glass without doping (CSA glass) does not emit PL after ultrafast laser irradiation as illustrated in Fig. 1(b). Typical broadband PL of Eu2+ ions arises in Eu: CSA glass after tailoring luminescence with fs laser. The FWHM of the emission spectrum reaches 190 nm, which is much larger than that of the reported value of ~90 nm in the previous reports30 (such as in the Eu-doped borate (Eu: BAN) glass (Fig. 1(c))). The anomalous spectra broadening can be attributed to the coexistence of Eu2+ ions and Eu3+ ions in glass (
Fig. S4(a)). Besides, the random distribution of Si and Al will enhance the structural disorder and asymmetry thus leading to a broad spectral band. Furthermore, the PL can be stimulated both by 375 nm and 405 nm resulting from the big centroid shift as well as the energy level splitting. The Eu-doped glasses usually have less absorption after 400 nm, the PL of Eu2+ excited by 405 nm laser is barely detectable in borate glass as shown in Fig. 1(c). However, by tailoring the optical property of Eu: CSA glass, the absorption in ultraviolet region of Eu2+ doped glass becomes higher (Fig. 1(d)), and the PL emission can be excited by 405 nm laser, which provides a new choice of excitation for data reading. Besides, the bandgap of Eu: CSA glass is approximately 3.65 eV according to Fig. 1(d), indicating three-photon absorption during laser-matter interaction. Consequently, reduction of Eu3+ and tailoring of optical properties inside glass are achieved simultaneously and allow for tuning the PL of the active ions. The distinct PL in Eu: CSA glass resulting from Eu3+ and Eu2+ exhibits the possibility to serve as an ODS medium. Besides, potential multi-application of rare earth ions-doped glasses has been proposed, and ODS technology is one of the most important demonstrations.
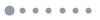
Figure 1.(a) PL spectra of initial Eu: CSA glass. (b) PL spectra of Eu: CSA and CSA glasses after irradiation. (c) PL spectra of Eu: BAN glass after ultrafast laser irradiation. The inset is the enlarged PL spectrum excited by 405 nm laser. (d) Absorption spectra of Eu: CSA and Eu: BAN glasses. Inset is an enlargement of absorbance from 350 to 430 nm.
To further demonstrate the performance of ODS in the devised glass, we systematically investigated the impact of used laser parameters including duration, pulse energy, repetition rate, and pulse number. As illustrated in Fig. 2(a), groups of dots are directly written into Eu: CSA glass by single pulse fs laser with controlled pulse durations and pulse energies. Reading out of dot-array is exhibited in Fig. 2(b) and the result indicates even single pulse can induce local material modifications with PL strong enough to be used for data storage. Figure 2(c) shows that the diameter of the written dots increases with the increase in the pulse energy. The size of written dots can be reduced to 600 nm when the pulse energy is reduced to 96 nJ (
Fig. S5(b)), and the density of ODS is approximately 1.4 Tbit cm–3. Figure 2(d) demonstrates the PL intensity of dots written with different pulse energies and pulse durations. The PL intensity increases with the increment in the writing pulse energy. A decrease in pulse duration leads to an increase in the PL intensity of Eu2+ and a decrease in the pulse energy threshold for writing emissive dots. Figure 2(e) demonstrates the PL intensity as a function of repetition rate and pulse number, which indicates the presence of a broad grayscale of PL intensity that can serve as another degree of freedom in ODS. Figure 2(f) demonstrates up to 16 levels of grayscales and in this case each written dot can be coded in a 4-bit-format (from 0000 to 1111), which allows further expansion of storage capacity. Dots in a 4-bit format can also be achieved by controlling pulse energy, as shown in
Fig. S6. It is worth to note the diameter of writing dots increases with the increase of pulse number and pulse energy. Too small or large bit spacing will lead to a decrease in bit contrast or storage density, as a result, appropriate bit spacing is very crucial to keep the balance between low cross-talk and high storage capacity.
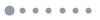
Figure 2.(a) Optical image of recorded information in the glass. (b) Reading out of information by PL mapping. Scale bar: 10 µm. (c) Relationship between emissive dot size and pulse energy. (d) PL intensity of dots written with diverse pulse durations and pulse energies. (e) PL intensity of dots written with various repetition rates and pulse numbers. (f) 16 levels of grayscale assignment based on the relation between PL intensity and pulse number.
As demonstrated in Fig. 3(a–c), Chinese characters of “zhe jiang da xue” are translated into a binary symbol and recorded in various layers of the transparent Eu: CSA glass. The dots in layer 1 and layer 2 are written by single pulse of fs laser with pulse energy of 200 nJ, and dots in layer 3 and layer 4 are written by 250 nJ and 300 nJ fs laser, respectively. The first layer of dots is written at the depth of 50 µm, and the spacing between adjacent layers in the z-direction is 10 µm. Reading out of the recorded information can be achieved by collecting the PL from the dots without and with fs laser writing, which corresponds to the “0” and “1” states in the binary system, respectively. No signal crosstalk is observed between different adjoining layers by controlling the writing pulse number and pulse energy (Fig. 3(b)) and it is also essential for 3D ODS. As the information writing is realized by single pulse incidence, the amount of data written per unit time can be considerably large, which is determined by the laser repetition rate and scanning speed of the stage. Therefore, high repetition frequency in the megahertz range is more favorable for improving information storage efficiency. Besides, a complicated logo pattern is also successfully recorded in glass by selectively tailoring the luminescence with continuous line scanning with 0.5 µJ pulse energy fs laser and the scanning speed is 80 µm/s. The recorded pattern can be read by 405 nm laser stimulation as demonstrated in Fig. 3(d) and it can also be stored in different layers. The non-uniformity of PL intensity, such as the low PL intensity in the bottom right, is due to the non-uniformity of glass thickness, which can be optimized by better polishing. The successful demonstration of 3D information recording and reading demonstrates the feasibility of ODS technology in the Eu2O3 doped aluminosilicate glass.
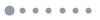
Figure 3.Optical information writing and readout. (a) Schematic diagram of 3D information writing in the glass. (b) Recorded information in glass in different layers. Dots in layer 1 and layer 2 are written by single pulse with 200 nJ. Dots in layer 3 and layer 4 are written by single pulse with 250 nJ and 300 nJ, respectively. Scale bar: 10 µm. (c) Encode information by binary format. (d) Complicated logo pattern recorded in the glass. Scale bar: 20 µm.
A long-term ODS technology with lifetime longer than a few hundred years can not only significantly reduce the energy dissipation associated with frequent data migration but also increase the savings in expenditures, including electricity and costs of storage devices. Consequently, it is necessary to examine the lifetime of recorded information in the devised material. As illustrated in Fig. 4(a), the stored information in Eu: CSA glass can survive under the temperature of up to 970 K. According to Arrhenius law (
Eq. S7), the lifetime (Fig. 4(b)) of the PL signal that can sustain the stability and readability of recorded information is evaluated up to 2×107 years which is several orders of magnitude higher than current PL-based ODS approaches (Fig. 4(c))6, 8. The temperature tolerance of other Eu2O3 doped aluminosilicate glasses (Eu: DSA, D represents MgO, CaO, SrO, BaO) is demonstrated in Fig. 4(d), after two-hours heating at 770 K, the PL intensity decreased by only 17%, indicating a generally excellent storage lifetime in similar glass systems. This is because that in Eu: DSA glass, the tetrahedral symmetry around Eu2+ will decrease with the incorporation of Al3+ and Si4+ sites, and the energy level splitting of 5d band increases. Consequently, the activation energy for thermal quenching becomes higher, resulting in a more stable PL. Notably, the recorded information in Eu: CSA glass can withstand highly intense ultraviolet irradiation (~100 kW/cm2) and be accurately read with an excitation power as low as 6 nW (corresponding to 2 W/cm2 in Fig. 4(e)). The decay rate of PL intensity is reduced significantly in Eu: CSA glass as compared with the tolerability of ultraviolet irradiation in Ag-doped silicate (Ag: CSA) glass and Eu: BAN glass (Fig. 4(f)). These results firmly confirm the great potential of the Eu: CSA glass-mediated ODS in highly robust long-term information storage.
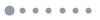
Figure 4.Stability of recorded information.(a) Thermal stability of PL in Eu: CSA glass. (b) Arrhenius plot of the decay rate. Grey area: error estimates. (c) Comparison of lifetime in ODS based on different media6, 8. (d) Comparison of thermostability of PL in Eu: DSA glasses. (e) PL stability of written dots under various excitation power densities. (f) PL stability of different ODS media.
In addition, the tailoring of luminescence can be universally attained in more glasses, such as employing other alkaline earth metal ions (including Mg, Sr, and Ba) to serve as glass modifiers. Similar broadband PL emitting by Eu2+ and several characteristic sharp PL peaks emitting by Eu3+ are collected, as shown in Fig. 5(a) and 5(b). It is worth noting that the emission of Eu2+ depends on surrounding environment. Hence by regulating the alkaline earth metal ions in aluminosilicate glasses, the PL emitted by Eu2+ ions can be tailored and it is flexibly tunable among the whole visible region. The Commission International de L’Eclairage (CIE) chromaticity coordinates of PL spectra in these aluminosilicate glasses changed gradually from (0.29, 0.35) to (0.43, 0.42) with an increase in the ratio of alkaline earth metal ion (Fig. 5(c)). As shown in Fig. 5(d), the energy gap between the 4f ground state and the lowest 5d excited level (Efd (free)) is approximately 4.216 eV (equal to 34000 cm–1) for the free Eu2+ ion36, 37. However, the actual 4f–5d energy position (Efd (A)) is generally smaller than that of the free 4f–5d energy gap when Eu2+ ions are doped into the host due to the influence of the surrounding environment on the 5d energy level. Generally, the emission of europium doped luminescence materials is determined by the spectroscopic redshift of the 5d energy position compared with that of free Eu2+ ion
consisting of the centroid shift (
) and the energy level splitting
37. The emission spectrum is influenced by the lowest 5d energy level and Stokes shift (∆S (A)). The Stokes shift is related to equilibrium position offset and phonon energy. In Eu: DSA glasses, a larger cation ratio results in a bigger spectroscopic redshift, which originates from the synergistic contribution of a large centroid shift, energy level splitting and Stokes shift as illustrated in Fig. 5(e). Typically, when the radius of alkaline earth metal ions increases, the spectral polarizability of the closest anion neighbors increases, the typical coordination number of cations also exhibits an increasing trend, and the distance between Eu2+ and O2– also decreases, hence leading to a bigger centroid shift. Thus, the luminescence properties of dopants in solid matrix can be manipulated in a wide range, which can greatly expand the potential applications of aluminosilicate glasses in storage, imaging, and display.
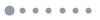
Figure 5.(a) PL spectra of dots written in the aluminosilicate glasses by fs laser. (b) PL spectra of initial glasses. Excitation wavelength: 375 nm. (c) Optical emission of Eu2+ in CIE coordinates. (d) Schematic diagram of the real 5d energy position in an inorganic solid. (e) Spectroscopic redshift in Eu: DSA glass.
Conclusions
Here, a stable ODS technique with an ultralong lifetime of 2×107 years is proposed by ultrafast laser selectively regulating the valence state of doped active ions and tailoring optical properties of Eu2+ ions inside the Eu-doped aluminosilicate glasses. It is demonstrated that the stored information exhibits excellent tolerability to temperature (970 K) and ultraviolet irradiation (100 kW/cm2). Furthermore, the active Eu2+ ions emit a broadband PL with FWHM of 190 nm, and the PL can be flexibly tuned in the entire visible range by adjusting the modifiers in glasses. The proposed ODS technique with ultralong lifetime is of great significance for breaking the bottleneck of large energy consumption in practical applications.