Author Affiliations
1School of Physics and Optoelectronic Engineering, Xidian University, Xi’an 710071, China2Beijing National Laboratory for Condensed Matter Physics, Institute of Physics, Chinese Academy of Sciences, Beijing 100190, China3Key Laboratory of Transparent and Opto-functional Inorganic Materials, Shanghai Institute of Ceramics,Chinese Academy of Sciences, Shanghai 201800, China4School of Physics Science and Engineering, Institute for Advanced Study, Tongji University, Shanghai 200092, Chinashow less
Abstract
We experimentally demonstrated a diode-pumped Kerr-lens mode-locked femtosecond laser with a Yb:CaYAlO4 crystal as the gain medium. Pulse duration as short as 33 fs was obtained directly from the oscillator at a repetition rate of 115 MHz. The central wavelength was at 1059 nm with a spectral bandwidth of 49 nm. These are, to the best of our knowledge, the shortest pulses generated from a Yb:CaYAlO4 oscillator.1. INTRODUCTION
In recent years, there has been great interest in the development of diode-pumped all-solid-state femtosecond lasers for wide applications in scientific researches and industries. Among them, -doped materials are highly suitable for ultrafast laser generation because of high quantum efficiency, large emission bandwidth, and freedom from deleterious effects such as excited state absorption, upconversion, and concentration quenching. As the most important advantage, the absorption bands of the -doped materials match well with the emission wavelengths of the commercially available high-brightness InGaAs laser diodes, which allows for building compact and efficient diode-pumped mode-locked femtosecond solid-state lasers.
Diode-pumped Yb lasers with sub-50-fs duration are desired for ultrahigh resolution optical coherent tomography; ultrafast spectroscopy; seeds for high-energy, high-repetition-rate terawatt laser systems; and so on. To realize such short pulse duration, a flat, smooth, and broad gain cross section of media is a prerequisite. Several kinds of -doped laser materials have been studied to generate sub-50-fs pulses. In 2006, Zaouter et al. realized a 47 fs passively mode-locked (Yb:CALGO) laser [1]. Pumped by a 350 mW single-mode laser diode, Agnesi et al. reported a passively mode-locked Yb:CALGO laser with 40 fs pulses and 20 nm wavelength tunable range [2]. In 2011, 35 fs pulses were produced from a Yb:YAG crystal and a (Yb:YCOB) crystal by virtue of Kerr-lens mode-locking (KLM) [3,4]. Most recently, a pure KLM Yb:CALGO oscillator was realized delivering 40 fs pulses with average power up to the watt level or shorter pulse duration down to 32 fs pumped by a polarized single-mode fiber laser [5].
As a promising laser host, (CYA) crystal has the same structure as CALGO crystal, belonging to the tetragonal compounds with the structure [6]. In CYA crystal, the octahedrons formed a perovskite-type structure, and the Ca ions are similar to the Y ions in size and randomly distributed around the Y cations [6]. In addition, the distance between the central metal ion and its ligand ions in the CYA crystal is as small as 0.19 nm, which causes the crystal field splitting in CYA crystal to be much larger than in most oxides [7]. As a result, both random replacement and a large split crystal field together lead to broadening of the spectrum of doping ions (, , , ) in the CYA crystal, which makes it possible to generate femtosecond pulses. Therefore, the CYA crystal is an interesting crystalline host for a mode-locking laser. Up to now, many rare-earth-doped CYA crystals, such as Nd:CYA [8,9], Yb:CYA [10,11], Tm:CYA [12], Er:CYA [8,13,14], and Ho:CYA [15], have been developed, and the thermal, spectroscopic, and laser characteristics have been extensively investigated. Among them, crystal as a laser gain medium with properties similar to that of Yb:CALGO has attracted the most attention. Because of the random distribution and the charge difference of the and ions, Yb:CYA has large inhomogeneous broadening of the emission spectrum. The full width at half-maximum (FWHM) fluorescence spectral bandwidth of the Yb:CYA crystal is 77 nm at σ polarization, and the fluorescence lifetime was measured to be 426 μs [16]. The thermal conductivity of the Yb:CYA crystal reached and along the and axes, respectively, while the thermal conductivity of pure CYA crystal was and , respectively [16]. In 2011, laser pulses of 156 fs with an average output power of 740 mW were generated from a diode-pumped Yb:CYA oscillator [17]. Dissipative soliton operation of a Yb:CYA laser was also reported with 7.4 ps uncompressed pulses and 340 fs compressed ones [18]. Benefiting from the broad FWHM fluorescence spectral bandwidth, it is expected that sub-50-fs pulses could be generated. Recently, Pirzio et al. reported a single-mode fiber-coupled 400 mW laser diode pumped femtosecond mode-locked Yb:CYA laser with semiconductor saturable absorber mirror, generating the shortest pulses of 43 fs and a wavelength tunability of 40 nm [19].
Sign up for Photonics Research TOC. Get the latest issue of Photonics Research delivered right to you!Sign up now
In this paper, we report on a diode-pumped Kerr-lens mode-locked femtosecond Yb:CYA laser. By enhancing the Kerr-lens nonlinearity and fine intracavity chirp compensation, laser pulses as short as 33 fs were generated directly from the output of the oscillator. The FWHM spectral bandwidth of the KLM pulses was 49 nm at the central wavelength of 1059 nm. To the best of our knowledge, this is not only the first demonstration of KLM operation in a diode-pumped Yb:CYA laser but also one of the shortest pulses directly generated from the diode-pumped bulk lasers.
2. EXPERIMENT SETUP
Figure 1 shows the schematic of the experimental setup. The Yb:CYA crystal used in our experiment was grown by the Czochralski technique and weakly doped with 2 at.% ions; it was cut along the c-direction with an aperture of and a thickness of 2 mm. To move the heat accumulation and keep a stable temperature, the uncoated crystal was wrapped with indium foil and mounted on a heat sink kept at 14°C and located in the middle of a standard -type cavity between two curved mirrors (M1, M2) with 75 mm radii of curvature (ROCs). To eliminate the surface reflection loss, the Yb:CYA crystal was placed at Brewster´s angle. The folding angle of the two arms is set to be 9.5° in order to compensate for the astigmatism of the cavity. Based on the ABCD matrix calculation, the laser beam waist at the focal spot in the crystal was about in the continuous wave (CW) operation. In one arm of the cavity, a high reflection (HR) mirror acted as the end mirror and was fixed on a translation stage. The other arm contained a pair of SF6 prisms (P1, P2) for chirp compensation and was terminated by an output coupler (OC) with transmittance of 0.5% (1020–1100 nm). Two arms of the cavity were of almost equal length, and the total cavity length was about 1.30 m, corresponding to a repetition rate of 115 MHz.

Figure 1.Experimental setup of the diode-pumped Kerr-lens mode-locked femtosecond Yb:CYA laser. M1 and M2, concave mirrors with ROC of 75 mm; HR, high reflection mirror; OC, plane output mirror; P1 and P2, SF6 prisms. Lower pictures show the beam profiles for CW and KLM captured after the OC.
We used a fiber-coupled laser diode (Jenoptik, JOLD-7.5-BAFC-105) as the pump laser with a maximum power of 7.5 W at 976 nm. The coupled fiber was designed with a 50 μm core diameter with a numerical aperture of 0.22. The pump beam from the fiber was reimaged into the crystal by a coupling system with a magnification of 1:0.8. The beam waist was measured by the knife-edge method to be around 20 μm, which well matched that of the laser mode in the crystal for effectively enhancing the KLM effect. The nonlasing absorption coefficient was measured to be about 38% at the pump power.
3. EXPERIMENT RESULT
In our setup, after passing through the coupling system and the half-inch concave mirror M1, the pump power incident in the crystal was 3.26 W, while the absorbed pump power was 1.24 W. Under this pump condition, we first aligned the laser cavity to generate the maximum output power for the CW operation. By using a 2.5% transmission output coupler, the maximum average output power was about 227 mW under the absorbed pump power of 1.27 W, corresponding to a slope efficiency of 36.6%. To achieve KLM, we replaced the output coupler with a 0.5% one to increase the intracavity laser intensity. In this case, the maximum average output power was 77 mW at the wavelength of 1051 nm. We carefully adjusted the position of the M2 mirror to the stability edge of the cavity. At this point, the average output power dropped to 25 mW while in this case the lasing wavelength became disordered in the 1030–1060 nm range. Then the KLM operation was initiated by a slight translation of the HR mirror. Once mode-locked, the average output power raised to 36 mW, and the mode-locking spectrum became smooth and flat. The mode-locking can be stably and continuously operated for at least 1 h in an open environment without resisting the air fluctuation. Although the KLM was mainly stopped by air flow and mechanical vibration, it can be restarted by slightly translating the end mirror. Figure 2 shows the KLM pulse train detected by a fast photodiode and recorded with a digital phosphor oscilloscope with 500 MHz bandwidth (Tektronix DOP 3052). There was no visible -switching modulation of the mode-locked pulse train. The beam profiles in both CW and KLM operations were measured in the near field by a commercial beam profiler (BeamAnalyzer USB), which is shown in Fig. 1. The intensity diameter of the CW laser beam was about 1.34 mm along the and axes, while it was about 1.50 mm for the KLM laser beam. The KLM beam diameter was a little larger than that of the CW beam. In both cases the laser runs in the fundamental mode with near diffraction-limited beam quality.
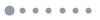
Figure 2.Mode-locked pulse trains at (a) 20 ns/div and (b) 2 ms/div, respectively.
In order to fully compensate for the intracavity dispersion, the tip-to-tip distance of the SF6 prism pair was set as 235 mm, introducing a group delay dispersion (GDD) of about at 1059 nm. Considering the normal GDD of about introduced by the 5 mm insertion of the prisms and the material dispersion of about by the Yb:CYA crystal, the net GDD in the cavity was about per round trip. The shortest pulse duration was obtained by precisely adjusting the insertion of the prism P2. Using a commercial intensity autocorrelator (APE: pulseCheck USB), we measured the autocorrelation trace of the shortest pulses, which is shown in Fig. 3(a). Assuming a pulse shape, the pulse duration was 33 fs. Figure 3(b) shows the corresponding spectrum of the KLM pulses. The mode-locked spectral bandwidth (FWHM) was as broad as 49 nm centered at 1059 nm. The spectral component over 1100 nm was not recorded due to the wavelength limitation of our spectrometer (wavelength range: 600–1100 nm; resolution: 0.4 nm.). The noise in the red tail in Fig. 3(b) was probably due to the low quantum efficiency of the Si detector used in our spectrometer. The time–bandwidth product of the pulses was 0.433, which is a little bigger than that of the Fourier transform-limited pulse, indicating there was still some uncompensated chirp in the cavity as well as that introduced by the 6.35 mm fused silica OC. For the approximate 30 fs pulses, higher order dispersion could not be completely compensated by the intracavity prism pair. The residual chirp could be further compensated by extracavity dispersion management by means of customized chirped mirrors, Gires–Tournois interferometer mirrors, prism pairs, or their combination. The 49 nm spectral bandwidth supports a Fourier transform-limited pulse duration of 24 fs. So there is great potential to generate sub-30-fs pulses from a diode-pumped Yb:CYA solid state laser.
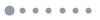
Figure 3.(a) Intensity autocorrelation trace and (b) laser spectrum of the KLM pulses from the Yb:CYA laser. The blue dotted and the red solid curves in (a) are the experimental data and the fitting, respectively.
4. CONCLUSION
In conclusion, we have demonstrated the first diode-pumped KLM femtosecond laser operation with Yb:CYA crystal. Pulses as short as 33 fs at the central wavelength of 1059 nm were directly generated from the oscillator. The pulse duration was very close to the shortest pulses ever produced from the Yb:CALGO laser (32 fs [5]). Considering the spectral bandwidth as broad as 49 nm, sub-30-fs pulses would be obtained by further extracavity dispersion compensation. The mode-locked pulse power was 36 mW for an absorbed pump power of 1.24 W. Power scaling up to 1 W is possible by replacing larger transmission output couplers and by good thermal management while keeping the short pulse duration. The compact, versatile diode-pumped solid state sub-30-fs laser may find important applications in many research fields.
ACKNOWLEDGMENT
Acknowledgment. This work is partially supported by the National Major Scientific Instruments Development Project of China (Grant No. 2012YQ120047) and the National Natural Science Foundation of China (Grant No. 61205130).
References
[1] Y. Zaouter, J. Didierjean, F. Balembois, G. Lucas Leclin, F. Druon, P. Georges, J. Petit, P. Goldner, B. Viana. 47-fs diode-pumped Yb3+:CaGdAlO4 laser. Opt. Lett., 31, 119-121(2006).
[2] A. Agnesi, A. Greborio, F. Pirzio, G. Reali, J. A. Au, A. Guandalini. 40-fs Yb3+:CaGdAlO4 laser pumped by a single-mode 350-mW laser diode. Opt. Express, 20, 10077-10082(2012).
[3] S. Uemura, K. Torizuka. Sub-40-fs pulses from a diode-pumped Kerr-lens mode-locked Yb-doped yttrium aluminum garnet laser. Jpn. J. Appl. Phys., 50, 010201(2011).
[4] A. Yoshida, A. Schmidt, V. Petrov, C. Fiebig, G. Erbert, J. Liu, H. Zhang, J. Wang, U. Griebner. Diode-pumped mode-locked Yb:YCOB laser generating 35 fs pulses. Opt. Lett., 36, 4425-4427(2011).
[5] P. Sévillano, P. Georges, F. Druon, D. Descamps, E. Cormier. 32-fs Kerr-lens mode-locked Yb:CaGdAlO4 oscillator optically pumped by a bright fiber laser. Opt. Lett., 39, 6001-6004(2014).
[6] C. F. Woensdregt, H. W. M. Janssen, A. Gloubokov, A. Pajaczkowska. Growth morphology of tetragonal ABCD4 compounds: theory and observations on Czochraski grown crystals. J. Cryst. Growth, 171, 392-400(1997).
[7] M. Yamaga, P. I. Macfarlane, K. Holliday, B. Henderson, N. Kodama, Y. Inoue. A study of substitutional disorder in Cr3+:CaYAlO4: I. fluorescence line narrowing. J. Phys., 8, 3487-3503(1996).
[8] E. F. Kustov, V. P. Petrov, D. S. Petrova, J. P. Udalov. Absorption and luminescence spectra of Nd3+ and Er3+ ions in monocrystals of CaYAlO4. Phys. Status Solidi A, 41, 379-383(1977).
[9] D. Li, X. Xu, S. Cheng, D. Zhou, F. Wu, Z. Zhao, C. Xia, J. Xu, J. Zhang, H. Zhu, X. Chen. Polarized spectral properties of Nd3+ ions in CaYAlO4 crystal. Appl. Phys. B, 101, 199-205(2010).
[10] P. O. Petit, J. Petit, P. Goldner, B. Viana. Inhomogeneous broadening of optical transitions in Yb:CaYAlO4. Opt. Mat., 30, 1093-1097(2008).
[11] D. Li, X. Xu, Y. Cheng, S. Cheng, D. Zhou, F. Wu, C. Xia, J. Xu, J. Zhang. Crystal growth and spectroscopic properties of Yb:CaYAlO4 single crystal. J. Cryst. Growth, 312, 2117-2121(2010).
[12] W. Wang, X. Yan, X. Wu, Z. Zhang, B. Hu, J. Zhou. Study of single-crystal growth of Tm3+:CaYAlO4 by the floating-zone method. J. Cryst. Growth, 219, 56-60(2000).
[13] J. A. Hutchinson, H. R. Verdum, B. H. T. Chai, B. Zandi, L. D. Merkle. Spectroscopic evaluation of CaYAlO4 doped with trivalent Er, Tm, Yb and Ho for eyesafe laser applications. Opt. Mater., 3, 287-306(1994).
[14] D. Zhou, X. Xu, X. Chen, H. Zhu, D. Li, J. Di, C. Xia, F. Wu, J. Xu. Crystal growth and spectroscopic properties of Er3+-doped CaYAlO4. Phys. Status Solidi A, 209, 730-735(2012).
[15] D. Zhou, J. Di, C. Xia, X. Xu, F. Wu, J. Xu, D. Shen, T. Zhao, A. Strzep, W. Ryba-Romanowski, R. Lisiecki. Spectroscopy and laser operation of Ho:CaYAlO4. Opt. Mater. Express, 3, 339-345(2013).
[16] D. Li, X. Xu, H. Zhu, X. Chen, W. Tan, J. Zhang, D. Tang, J. Ma, F. Wu, C. Xia, J. Xu. Characterization of laser crystal Yb:CaYAlO4. J. Opt. Soc. Am. B, 28, 1650-1654(2011).
[17] W. Tan, D. Tang, X. Xu, D. Li, J. Zhang, C. Xu, J. Xu. Femtosecond and continuous-wave laser performance of a diode-pumped Yb3+:CaYAlO4 laser. Opt. Lett., 36, 259-261(2011).
[18] W. Tan, D. Tang, C. Xu, J. Zhang, X. Xu, D. Li, J. Xu. Evidence of dissipative solitons in Yb3+:CaYAlO4. Opt. Express, 19, 18495-18500(2011).
[19] F. Pirzio, S. D. Di Dio Cafiso, M. Kemnizer, A. Guandalini, F. Kienle, S. Veronesi, M. Tonelli, J. A. der Au, A. Agnesi. Sub-50-fs widely tunable Yb:CaYAlO4 laser pumped by 400-mW single-mode fiber-coupled laser diode. Opt. Express, 23, 9790-9795(2015).